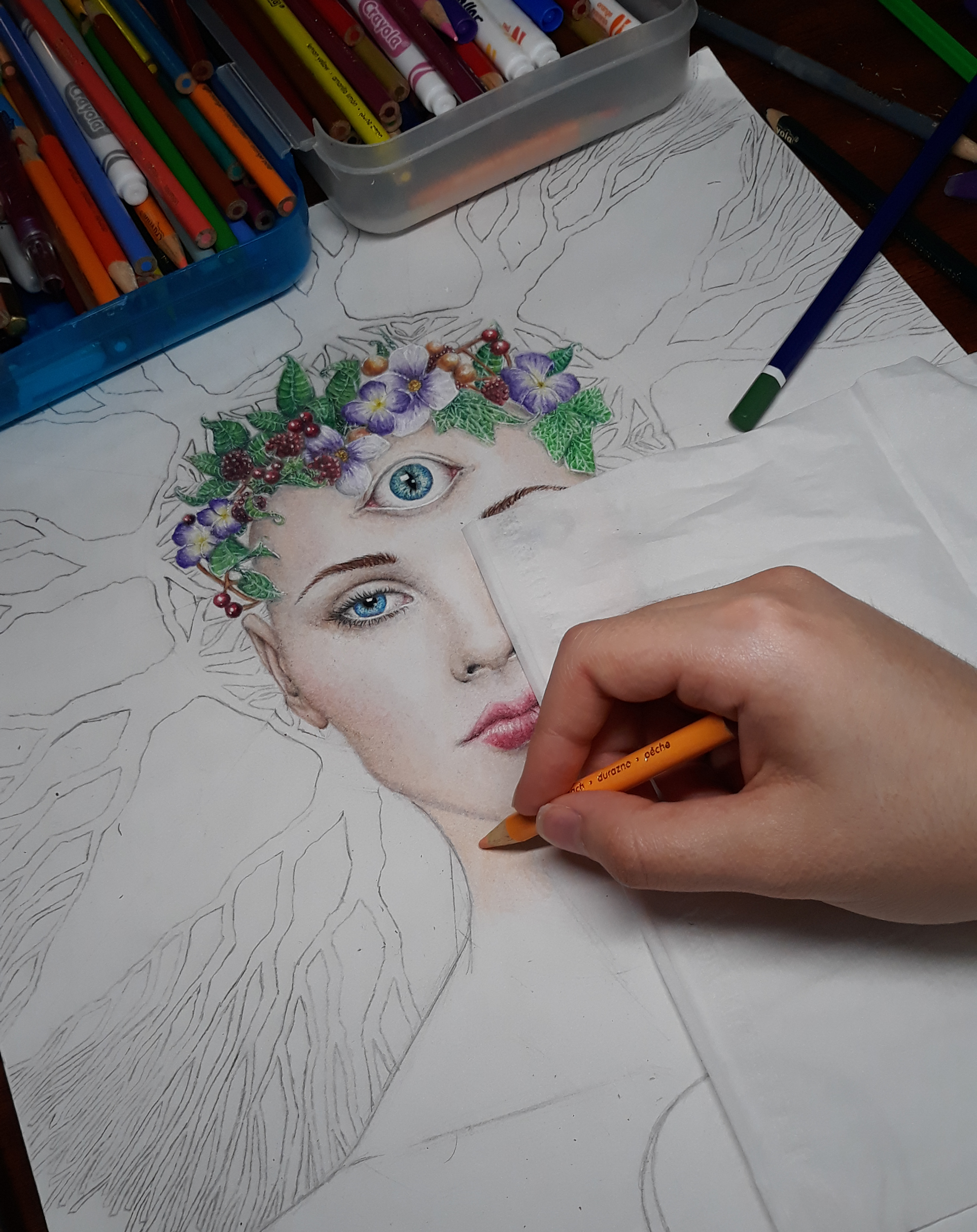
Computer science and molecular biology major Alexa Mallar ’27 has a passion for the visual, pursuing her love of art while also working as an undergraduate researcher in the Cheeseman Lab.
Mark Sullivan | Spectrum
June 4, 2025
“Visual art has been a passion and a core part of my identity since before attending MIT,” says Alexa Mallar ’27, a computer science and molecular biology major from Miami who is a recipient of the Norman L. Greenman (1944) Memorial Scholarship.
As an undergraduate researcher in the lab of Iain Cheeseman, MIT professor of biology and member of the Whitehead Institute, she helps develop computational tools for biological data analysis. Outside the lab, Mallar pursues her love of art, creating detailed graphite pieces in a hyperrealistic-surrealist style and experimenting with various media, including color pencil, charcoal, and multimedia sculpture, sharing her work on Instagram. Expanding her creative interests, she has explored 3-D printing through MIT MakerLodge, has taken 21T.101 Intro to Acting, and is taking 21W.756 Reading and Writing poetry in spring 2025. “Through visual and performing arts and creative writing, I continue to find new ways to express my creativity and grow as an artist,” she says.
What inspires you about creating art?
It’s a multitude of things. It’s a technical fascination with capturing details on a piece of paper and trying really hard to make it look like a photograph. There’s the enjoyment of the technical aspects of the task. There’s also an intellectual satisfaction that comes with creating art. I like incorporating surrealism into my work often because it lends itself to creating more visual meaning than a purely realistic piece would; there are several artists I follow and try to incorporate aspects of their work into mine, trying different things. There’s the experimental value of trying different media and artistic styles. I love exploring. I love expressing new ideas. Art is really a great way to do it.
Is there a connection between what you do as a scientist and as an artist?
The nature of my art is very visual, and I think about what I do in computer science or in research now in a very visual way. I map a diagram in my head of input and output. Anything I do is inherently visualized.
Sometimes the connection goes the other way—my interest in math and science bleeds into my art. Designing counterweights to balance sculptures or geometrically mapping out perspective and proportions are a few examples. I also love sneaking in little “easter eggs.” A few years ago, I created a piece featuring a woman with a third eye and a tree-branch crown, where the branching levels followed the Fibonacci sequence.
What is the story behind the mermaid drawings on your Instagram page?
“There’s an event every May called MerMay. Artists on Instagram will do successive drawings of different mermaids based on prompts. I wanted to join in, so I designed my own mermaid. I just started by imagining her face, and it evolved into her holding an orb I called the Eye of the Sea. It was really fun.”
After college, will you be pursuing both science and art?
“That’s a good question. I kind of have a 30-degree angle I’m heading in, not a specific path. I know that I will keep drawing in my free time, and the creative thinking and visualization skills will bleed into any other part of my work that I do, whether that be in computer science or research. Maybe designing a front end is where my creative spirit will contribute to the computer science work that I do.
“I plan to work for Amazon [in summer 2025], having received a return offer after working there last summer. I’m getting a sense of the different environments I could go to. If I can find a way to combine [art and career] I will. I’ll find a way to do as many things as I can that interest me.”
How has your MIT experience helped you on your path?
“It has been an amazing resource. MIT offers so many different classes and interdisciplinary opportunities. I was able to explore entrepreneurship through the Martin Trust Center at MIT, enrolling in the Undergraduate Engineering Entrepreneurship Certificate program. That’s one avenue I wouldn’t have been able to explore otherwise without MIT. Acting is not something I would have even tried before having the opportunity to do it at MIT. I’m rediscovering my love for creative writing through classes at MIT, and I’m really enjoying it. If I hadn’t been able to fit a poetry workshop into my class schedule, I probably wouldn’t be writing nearly as much this semester. I’m really glad I have that opportunity.
“MIT is in an amazing spot for someone in my specific major, with the huge presence of biotech in Cambridge. This is an optimal place for both computer science and biological research. We have the Whitehead Institute, Pfizer, Moderna, all within walking distance of campus. There’s a lot to explore, an intersection of interests, and I really appreciate that is available to me at MIT.”