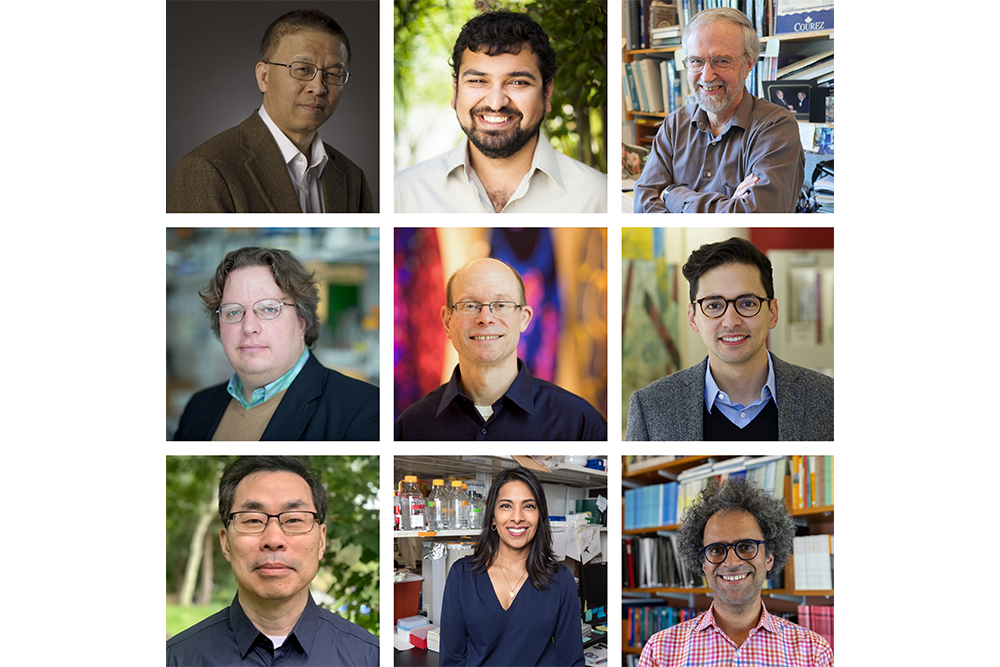
Tenth anniversary of the program rewards three innovative projects.
Aaron Braddock | Office of the Provost
June 13, 2022
MIT Provost Cynthia Barnhart has announced three Professor Amar G. Bose Research Grants to support bold research projects across diverse areas of study including biology, engineering, and the humanities.
The three grants honor the visionary and bold thinking in the winning proposals of the following nine researchers: John J. and Dorothy Wilson Professor of Health Sciences and Technology and Electrical Engineering and Computer Science Sangeeta Bhatia; Carl Richard Soderberg Professor of Power Engineering Gang Chen; professor of biology Jianzhu Chen; associate professor of biology Michael Hemann; professor of anthropology and Margaret MacVicar Faculty Fellow Graham Jones; Latham Family Career Development Professor Sebastian Lourido; assistant professor of computer science Arvind Satayanaryan; Howard Hughes Medical Institute Professor Graham Walker; and David H. Koch Professor in Science Michael Yaffe;
“Innovation is born when a unique vision drives daring researchers to take on risky and adventurous projects, a notion that Amar Bose understood well,” says Barnhart. “With support and recognition from this program, these nine talented and forward-thinking faculty have the freedom to explore and study areas not typically backed by conventional funding sources.”
The program was named for the visionary founder of the Bose Corporation and MIT alumnus, Amar G. Bose ’51, SM ’52, ScD ’56. After gaining admission to MIT, Bose became a top math student and a Fulbright Scholarship recipient. He spent 46 years as a professor at MIT, led innovations in sound design, and founded the Bose Corporation in 1964. MIT launched the program a decade ago.
“The legendary explorations and innovations of Professor Amar Bose inspire the Bose Research Grant program,” says President Emerita and Professor Susan Hockfield. “The grants support projects that reach beyond the horizon and so would not receive funding from standard sources. Since its inception, the program has supported 49 MIT faculty to pursue their most compelling ideas and, in doing so, to join the Bose Fellows community of like-minded adventurers.”
The program, which has honored 35 projects to date, is a tribute to the legacy of Bose, who believed that passion and curiosity drive innovation. With that spirit in mind, the projects typically supported by the program are original, cross-disciplinary, and high-risk. The program has encouraged collaborative projects, as reflected in this year’s winners.
This year’s recipients are:
Gang Chen of the Department of Mechanical Engineering. With his proposal, “Photomolecular Effect and Clouds Thinning,” Chen will advance research into his discovery of a way in which photons can be absorbed by cleaving off water clusters from the water-air surface, significantly impacting technologies related to energy and water and climate models.
Graham Jones of the Anthropology Section and Arvind Satayanaryan of the Department of Electrical Engineering and Computer Science (EECS). Their “Magical Data Visualization” proposal uses performance magic to create new visualizations that are responsive to the users’ intent, potentially impacting how misinformation spreads.
Graham Walker, Michael Hemann, Michael Yaffe, Sebastian Lourido, Jianzhu Chen of the Department of Biology and Sangeeta Bhatia of EECS and the Institute of Medical Engineering and Science. Their proposal, “Addressing Critical Human Health Problems with a Special Heme-binding Peptide,” uses a recently discovered plant peptide that binds and sequesters a molecule critical in hemoglobin oxygen binding in a new way, which has significant implications on many health issues.
“This year, more than a dozen faculty members from departments across all five schools and the college participated in the evaluations,” says Chancellor for Academic Advancement Eric Grimson. “Their diverse perspectives were critical in assessing what was a very strong field of interesting proposals. We are grateful for their generous commitment of time and energy and the thoughtfulness with which they approached the selection process.”
The program explores out-of-the-box ideas that would face difficulty in acquiring funding through traditional means but have the potential for strong impacts on the scientific community. Any member of the faculty in any discipline in MIT’s five schools and college is eligible to submit a proposal for a Bose Research Grant, which provides funding over three years.