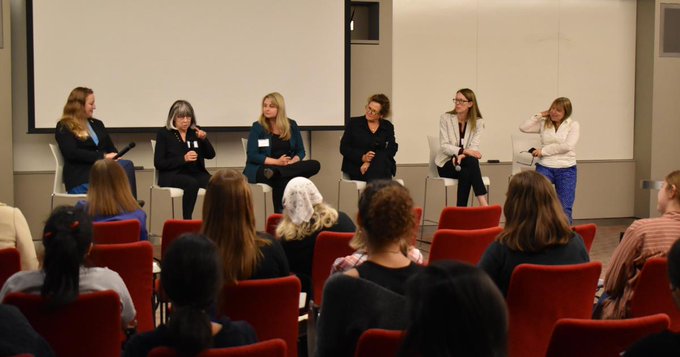
Mixing joy and resolve, event celebrates women in science and addresses persistent inequalities
David Orenstein | The Picower Institute for Learning and Memory
October 2, 2024
The Kuggie Vallee Distinguished Lectures and Workshops presented inspiring examples of success, even as the event evoked frank discussions of the barriers that still hinder many women scientists.
For two days at The Picower Institute for Learning and Memory at MIT, participants in the Kuggie Vallee Distinguished Lectures and Workshops celebrated the success of women in science and shared strategies to persist through, or better yet dissipate, the stiff headwinds women still face in the field.
“Everyone is here to celebrate and to inspire and advance the accomplishments of all women in science,” said host Li-Huei Tsai, Picower Professor in Brain and Cognitive Sciences and director of The Picower Institute, as she welcomed an audience that included scores of students, postdocs and other research trainees. “It is a great feeling to have the opportunity to showcase examples of our successes and to help lift up the next generation.”
Tsai earned the honor of hosting the event after she was named a Vallee Visiting Professor in 2022 by the Vallee Foundation. Foundation President Peter Howley, a professor of pathological anatomy at Harvard, said the global series of lectureships and workshops were created to honor Kuggie Vallee, a former Lesley College Professor who worked to advance the careers of women.
During the program Sept. 24-25, speakers and audience members alike made it clear that helping women succeed requires both recognizing their achievements and resolving to change social structures in which they face marginalization.
Inspiring achievements
Lectures on the first day featured two brain scientists who have each led acclaimed discoveries that have been transforming their fields.
Michelle Monje, a pediatric neuro-oncologist at Stanford whose recognitions include a MacArthur Fellowship, described her lab’s studies of brain cancers in children, which emerge at specific times in development as young brains adapt to their world by wiring up new circuits and insulating neurons with a fatty sheathing called myelin. Monje has discovered that when the precursors to myelinating cells called oligodendrocyte precursor cells harbor cancerous mutations, the tumors that arise—called gliomas—can hijack those cellular and molecular mechanisms. To promote their own growth, gliomas tap directly into the electrical activity of neural circuits by forging functional neuron-to-cancer connections, akin to the “synapse” junctions healthy neurons make with each other. Years of her lab’s studies, often led by female trainees, have not only revealed this insidious behavior (and linked aberrant myelination to many other diseases as well), but also revealed specific molecular factors involved. Those findings, Monje said, present completely novel potential avenues for therapeutic intervention.
“This cancer is an electrically active tissue and that is not how we have been approaching understanding it,” she said.
Erin Schuman, who directs the Max Planck Institute for Brain Research in Frankfurt and has won honors including the Brain Prize, described her groundbreaking discoveries related to how neurons form and edit synapses along the very long branches—axons and dendrites—that give the cells their exotic shapes. Synapses form very far from the cell body where scientists had long thought all proteins, including those needed for synapse structure and activity, must be made. In the mid-1990s Schuman showed that the protein-making process can occur at the synapse and that neurons stage the needed infrastructure—mRNA and ribosomes—near those sites. Her lab has continued to develop innovative tools to build on that insight, cataloging the stunning array of thousands of mRNAs involved, including about 800 that are primarily translated at the synapse, studying the diversity of synapses that arise from that collection, and imaging individual ribosomes such that her lab can detect when they are actively making proteins in synaptic neighborhoods.
Persistent headwinds
While the first day’s lectures showcased examples of women’s success, the second day’s workshops turned the spotlight on the social and systemic hindrances that continue to make such achievements an uphill climb. Speakers and audience members engaged in frank dialogues aimed at calling out those barriers, overcoming them, and dismantling them.
Susan Silbey, Leon and Anne Goldberg Professor of Humanities, Sociology and Anthropology at MIT and Professor of Behavioral and Policy Sciences in the Sloan School of Management, told the group that as bad as sexual harassment and assault in the workplace are, the more pervasive, damaging and persistent headwinds for women across a variety of professions are “deeply sedimented cultural habits” that marginalize their expertise and contributions in workplaces, rendering them invisible to male counterparts, even when they are in powerful positions. High-ranking women in Silicon Valley who answered the “Elephant in the Valley” survey, for instance, reported high rates of many demeaning comments and demeanor, as well as exclusion from social circles. Even Supreme Court justices are not immune, she noted, citing research showing that for decades female justices have been interrupted with disproportionate frequency during oral arguments at the court. Silbey’s research has shown that young women entering the engineering workforce often become discouraged by a system that appears meritocratic but in which they are often excluded from opportunities to demonstrate or be credited for that merit and are paid significantly less.
“Women’s occupational inequality is a consequence of being ignored, having contributions overlooked or appropriated, of being assigned to lower status roles, while men are pushed ahead, honored and celebrated, often on the basis of women’s work,” Silbey said.
Often relatively small in numbers, women in such workplaces become tokens—visible as different but still treated as outsiders, Silbey said. Women tend to internalize this status, becoming very cautious about their work while some men surge ahead in more cavalier fashion. Silbey and speakers who followed illustrated the effect this can have on women’s careers in science. Kara McKinley, an assistant professor of stem cell and regenerative biology at Harvard, noted that while the scientific career “pipeline” is some areas of science is full of female graduate students and postdocs, only about 20 percent of natural sciences faculty positions are held by women. Strikingly, women are already significantly depleted in the applicant pools for assistant professor positions, she said. Those who do apply tend to wait until they are more qualified than the men they are competing against. McKinley and Silbey each noted that women scientists submit fewer papers to prestigious journals, with Silbey explaining that it’s often because women are more likely to worry that their studies need to tie up every loose end. Yet, said Stacie Weninger, a venture capitalist and president of the F-Prime Biomedical Research Initiative and a former editor at Cell Press, women were also less likely than men to rebut rejections from journal editors, thereby accepting the rejection even though rebuttals sometimes work.
Several speakers including Weninger and Silbey said pedagogy must change to help women overcome a social tendency to couch their assertions in caveats when many men speak with confidence and are therefore perceived as more knowledgeable.
At lunch, trainees sat in small groups with the speakers. They shared sometimes harrowing personal stories of gender-related difficulties in their young careers and sought advice on how to persist and remain resilient. Schuman advised the trainees to report mistreatment, even if they aren’t confident that university officials will be able to effect change, at least to make sure patterns of mistreatment get on the record. Reflecting on discouraging comments she experienced early in her career, Monje advised students to build up and maintain an inner voice of confidence and draw upon it when criticism is unfair.
“It feels terrible in the moment, but cream rises,” Monje said. “Believe in yourself. It will be OK in the end.”
Lifting each other up
Speakers at the conference shared many ideas to help overcome inequalities. McKinley described a program she launched in 2020 to ensure that a diversity of well-qualified women and non-binary postdocs are recruited for and apply for life sciences faculty jobs: the Leading Edge Symposium. The program identifies and names fellows—200 so far—and provides career mentoring advice, a supportive community, and a platform to ensure they are visible to recruiters. Since the program began, 99 of the fellows have gone on to accept faculty positions at various institutions.
In a talk tracing the arc of her career, Weninger, who trained as a neuroscientist at Harvard, said she left bench work for a job as an editor because she wanted to enjoy the breadth of science, but also noted that her postdoc salary didn’t even cover the cost of child care. She left Cell Press in 2005 to help lead a task force on women in science that Harvard formed in the wake of comments by then-president Lawrence Summers widely understood as suggesting that women lacked “natural ability” in science and engineering. Working feverishly for months, the task force recommended steps to increase the number of senior women in science, including providing financial support for researchers who were also caregivers at home so they’d have the money to hire a technician. That extra set of hands would afford them the flexibility to keep research running even as they also attended to their families. Notably, Monje said she does this for the postdocs in her lab.
A graduate student asked Silbey at the end of her talk how to change a culture in which traditionally male-oriented norms marginalize women. Silbey said it starts with calling out those norms and recognizing that they are the issue, rather than increasing women’s representation in, or asking them to adapt to, existing systems.
“To make change it requires that you do recognize the differences of the experiences and not try to make women exactly like men or continue the past practices and think, ‘Oh, we just have to add women into it’,” she said.
Silbey also praised the Kuggie Vallee event at MIT for assembling a new community around these issues. Women in science need more social networks where they can exchange information and resources, she said.
“This is where an organ, an event like this, is an example of making just that kind of change: women making new networks for women,” she said.