Author: Raleigh McElvery
October 30, 2021
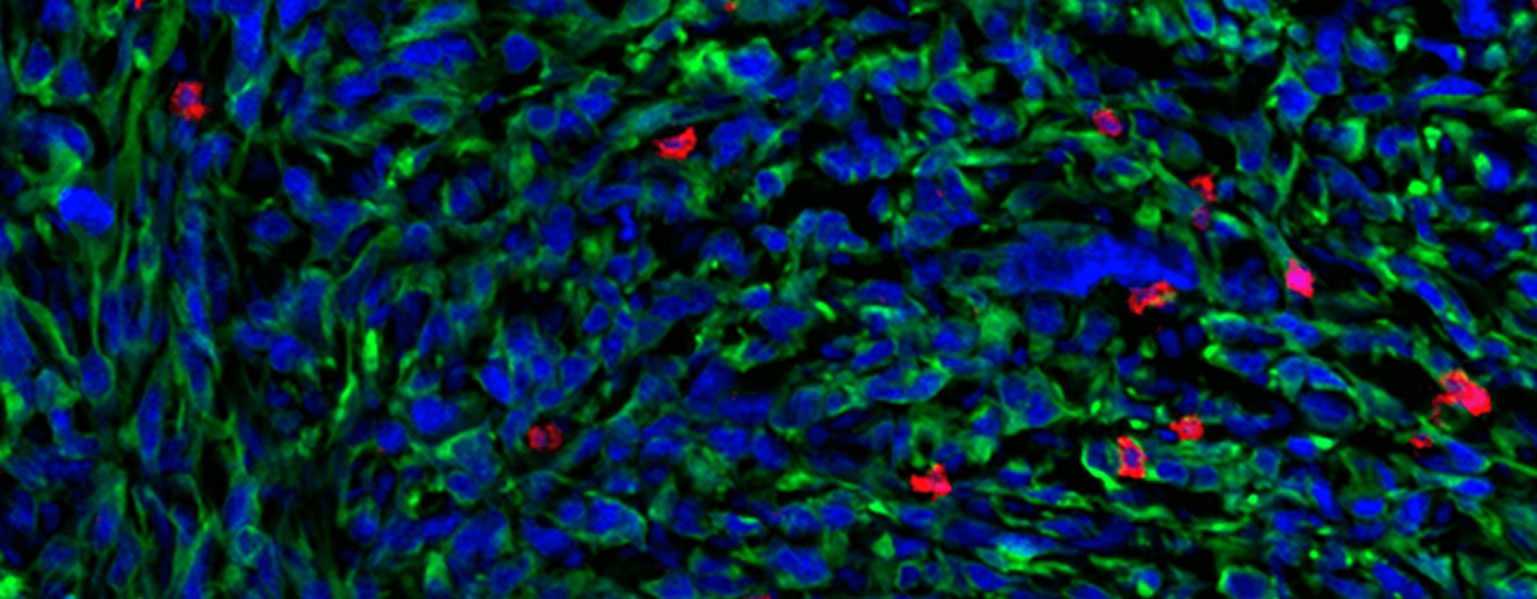
Researchers decipher when and why immune cells fail to respond to immunotherapy, suggesting that T cells need a different kind of prodding to re-engage the immune response.
Grace van Deelen
October 29, 2021
Non-small cell lung cancer (NSCLC) is the most common type of lung cancer in humans. Some patients with NSCLC receive a therapy called immune checkpoint blockade (ICB) that helps kill cancer cells by reinvigorating a subset of immune cells called T cells, which are “exhausted” and have stopped working. However, only about 35% of NSCLC patients respond to ICB therapy. Stefani Spranger’s lab at the MIT Department of Biology explores the mechanisms behind this resistance, with the goal of inspiring new therapies to better treat NSCLC patients. In a new study published on Oct. 29 in Science Immunology, a team led by Spranger lab postdoc Brendan Horton revealed what causes T cells to be non-responsive to ICB — and suggested a possible solution.
Scientists have long thought that the conditions within a tumor were responsible for determining when T cells stop working and become exhausted after being overstimulated or working for too long to fight a tumor. That’s why physicians prescribe ICB to treat cancer — ICB can invigorate the exhausted T cells within a tumor. However, Horton’s new experiments show that some ICB-resistant T cells stop working before they even enter the tumor. These T cells are not actually exhausted, but rather they become dysfunctional due to changes in gene expression that arise early during the activation of a T cell, which occurs in lymph nodes. Once activated, T cells differentiate into certain functional states, which are distinguishable by their unique gene expression patterns.
In order to determine why some tumors are resistant to ICB, Horton and the research team studied T cells in murine models of NSCLC. The researchers sequenced messenger RNA from the responsive and non-responsive T cells in order to identify any differences between the T cells. Supported in part by the Koch Institute Frontier Research Program, they used a technique called Seq-Well, developed in the lab of fellow Koch Institute member J. Christopher Love, the Raymond A. (1921) and Helen E. St. Laurent Professor of Chemical Engineering and a co-author of the study. The technique allows for the rapid gene expression profiling of single cells, which permitted Spranger and Horton to get a very granular look at the gene expression patterns of the T cells they were studying.
Seq-Well revealed distinct patterns of gene expression between the responsive and non-responsive T cells. These differences, which are determined when the T cells assume their specialized functional states, may be the underlying cause of ICB resistance.
Now that Horton and his colleagues had a possible explanation for why some T cells did not respond to ICB, they decided to see if they could help the ICB-resistant T cells kill the tumor cells. When analyzing the gene expression patterns of the non-responsive T cells, the researchers had noticed that these T cells had a lower expression of receptors for certain cytokines, small proteins that control immune system activity. To counteract this, the researchers treated lung tumors in murine models with extra cytokines. As a result, the previously non-responsive T cells were then able to fight the tumors — meaning that the cytokine therapy prevented, and potentially even reversed, the dysfunctionality.
Administering cytokine therapy to human patients is not currently safe, because cytokines can cause serious side effects as well as a reaction called a “cytokine storm,” which can produce severe fevers, inflammation, fatigue, and nausea. However, there are ongoing efforts to figure out how to safely administer cytokines to specific tumors. In the future, Spranger and Horton suspect that cytokine therapy could be used in combination with ICB.
“This is potentially something that could be translated into a therapeutic that could increase the therapy response rate in non-small cell lung cancer,” Horton says.
Spranger agrees that this work will help researchers develop more innovative cancer therapies, especially because researchers have historically focused on T cell exhaustion rather than the earlier role that T cell functional states might play in cancer.
“If T cells are rendered dysfunctional early on, ICB is not going to be effective, and we need to think outside the box,” she says. “There’s more evidence, and other labs are now showing this as well, that the functional state of the T cell actually matters quite substantially in cancer therapies.” To Spranger, this means that cytokine therapy “might be a therapeutic avenue” for NSCLC patients beyond ICB.
Jeffrey Bluestone, the A.W. and Mary Margaret Clausen Distinguished Professor of Metabolism and Endocrinology at the University of California-San Francisco, who was not involved with the paper, agrees. “The study provides a potential opportunity to ‘rescue’ immunity in the NSCLC non-responder patients with appropriate combination therapies,” he says.
This research was funded by the Pew-Stewart Scholars for Cancer Research, the Ludwig Center for Molecular Oncology, the Koch Institute Frontier Research Program through the Kathy and Curt Mable Cancer Research Fund, and the National Cancer Institute.
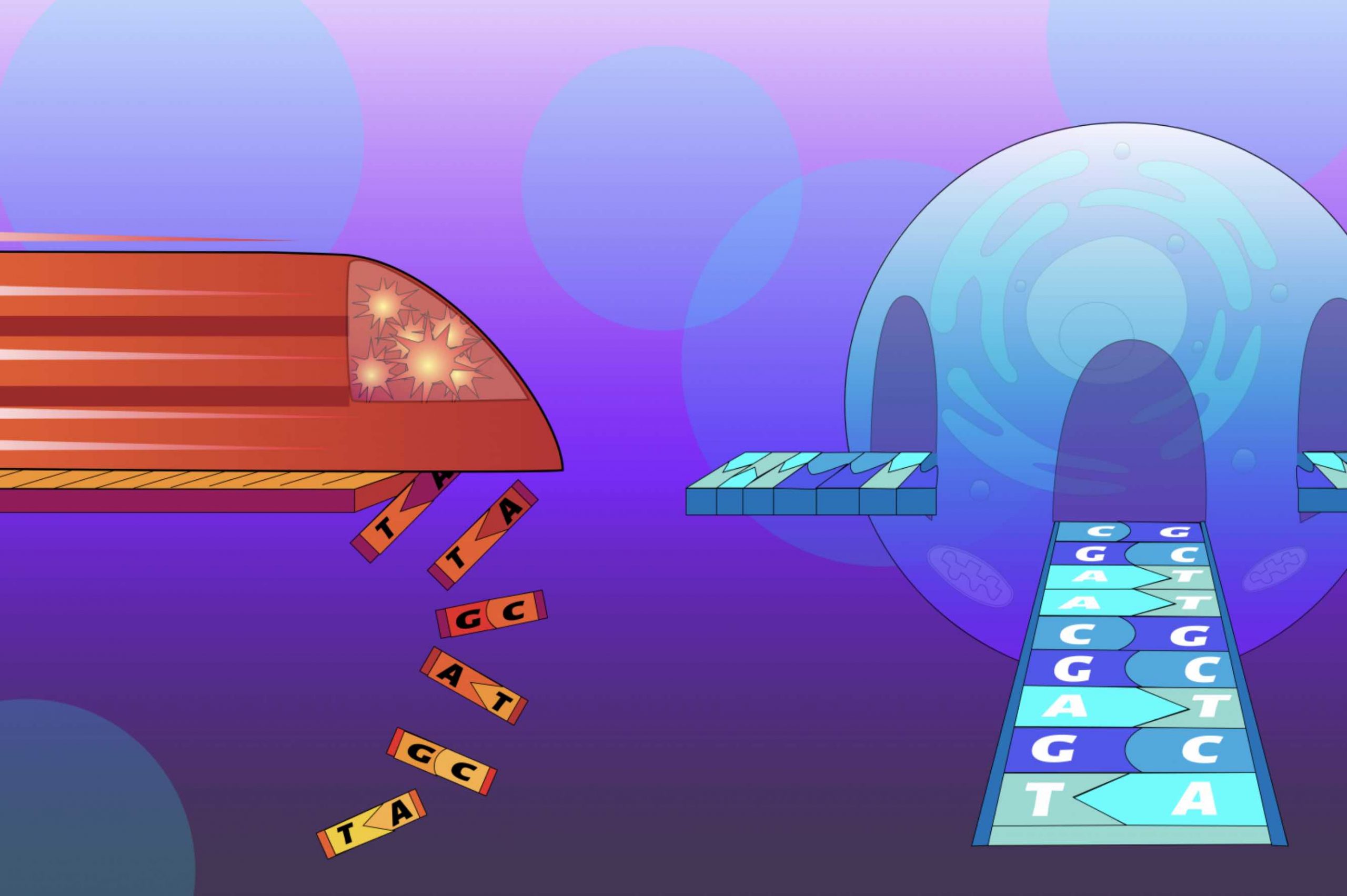
Eva Frederick | Whitehead Institute
October 25, 2021
Many people — around half of the adult population — are infected with a type of herpesvirus called human cytomegalovirus, or HCMV. Though mostly asymptomatic, the virus can be dangerous for immunocompromised people and unborn babies. Because HCMV is so widespread, the chance of a baby becoming infected in utero is around one in 200, and that infection can lead to problems with the baby’s brain, lungs and growth.
In a new paper from Whitehead Institute Member Jonathan Weissman published on October 25 in Nature Biotechnology, Weissman and colleagues turn cutting-edge CRISPR and single cell sequencing technologies on this virus, providing the most detailed picture yet on how viral and human genes interact to create an HCMV infection — and revealing new ways to potentially derail the virus’ progression through manipulating viral and host genes.
The research could provide an important road map for future studies of host-pathogen interactions, as well as inform antiviral drug design. Over the course of the project, the researchers generated a list of both viral and host genes that were either essential for the virus to replicate, or could potentially be manipulated to confer some immunity to the host cell. “Now that we have this list, we have a list of potential targets that one might now go ahead and develop drugs against,” said Marco Hein, the first author and a former postdoctoral researcher in the Weissman Lab.
Seeing both sides
Millions of years of evolution have created a complex web of interactions between virus and host. For example, viruses have their own set of genes, but they also depend on some human genes, called host factors. Hijacking these host factors allows the viruses to invade cells in the body and replicate their own genetic material.
Hein, who is now a researcher at the Chan Zuckerberg Biohub in San Francisco, and Whitehead Institute Member Jonathan Weissman, who is also a professor of biology at the Massachusetts Institute of Technology and Koch Institute and an investigator of the Howard Hughes Medical Institute, sought to gain a more thorough understanding of the web of host-viral interactions that arises throughout the course of an infection. “[We wanted to know] what actually happens when we [knock out or weaken] those critical factors,” Hein said. “Can we prevent infection? If so, what ‘goes wrong’ from the perspective of the virus?”
They chose HCMV as a test subject because, for one thing, the virus has a double-stranded DNA genome like humans. That means that CRISPR technologies that work by snipping DNA could theoretically work for both the virus and the host. “And because CMV is an important human pathogen and it’s such a complex and intricate virus, we thought we would have a chance to really discover something new,” said Weissman.
A series of screens
The researchers first set out, using a molecular technique called CRISPR screening, to determine whether any regions of the viral or host genomes in particular had an impact on the fate of infected host cells. By systematically knocking out individual genes in a large population of viruses and host cells, the researchers could then assess how essential each gene was to the infection.
The project took on a new dimension in 2016 with the development of accessible, large-scale single cell sequencing. “We had this idea to put together the CRISPR screening and the single cell sequencing, and [a screening method called PerturbSeq],” Hein said. “Basically, you perturb genes in a cell population and then you read out what happens to the cells, not just by measuring survival, but by actually looking at the pattern of gene expression in those cells over time.”
Combining these methods generated a huge set of data, which provided the researchers with a clearer view of which genes were important and when. “The single cell sequencing lets us watch the steps in the viral life cycle with much higher precision, and then the perturbation lets us understand how host and viral factors allow the virus to manipulate the host and complete its life cycle,” Weissman said.
The resultant data showed how the virus’ typical trajectory — from the initial waves of viral gene expression, to replication of the full viral genome, to the final step of budding off into newly-formed virions — could be derailed by altering specific viral genes. It also clued the researchers in to which host genes the virus depended on at what stages for a ‘successful’ infection.
“The course of infection is pre-programmed into the viral genome,” Hein said. “If you want to interfere with the course of infection you can do that by targeting a viral factor, or you can do it indirectly by targeting the host factor. And the outcomes are conceptually different. If you target a virus factor you derail the program that the virus would normally follow. If you target a host factor, the program itself is unchanged, but you change how far the virus gets in executing the program.”
These findings will be useful tools for the development of drugs that can be used as part of an antiviral “cocktail.” Because viruses and other pathogens are living creatures that can mutate and adapt to changing conditions, a common thread among antiviral treatments involves combining several drugs with different viral targets. This ensures the most complete eradication of viruses possible, reducing the chance that some will survive and create a new resistant population.
While the researchers’ list of essential viral genes provide parts of HCMV to target with drug cocktails, the list of contributing human genes could open the door for a more indirect therapy. “If you target a host factor to affect the virus, it’s much more difficult for the virus to escape because it can’t just mutate so the drug doesn’t bind anymore — it would have to mutate away from dependency on a host protein, which is much more complicated,” Hein said.
Of course, there are drawbacks to potentially targeting a human gene or protein to treat an infection, and much more work would need to be done for a viable treatment to emerge via this avenue of research. “If you target a host factor, you’re by definition targeting a protein that’s in our body, doing its normal job, so the risk of side effects is much higher,” Hein said.
Few drugs like this have made it past clinical trials; one famous example is hydroxychloroquine, which has been used successfully to treat malaria, and unsuccessfully to treat COVID-19.
In the future, Hein and Weissman hope to turn their multi-level approach for studying infection toward other viruses such as SARS-CoV-2. Although the novel coronavirus does not have double-stranded DNA that can be altered via CRISPR, the researchers can still investigate which host genes are essential at what stage of infection, and use their methods-driven approach to hopefully glean unexpected findings from a well-studied virus.
“I’m always driven by what technology can do,” Hein said. “I like to run a study in a systems-wide manner and then come up with some findings that you would have not found if you had only looked at one gene or protein at a time or looked at things more in the conventional way. This kind of high-level conclusion is what I personally always find the most exciting.”
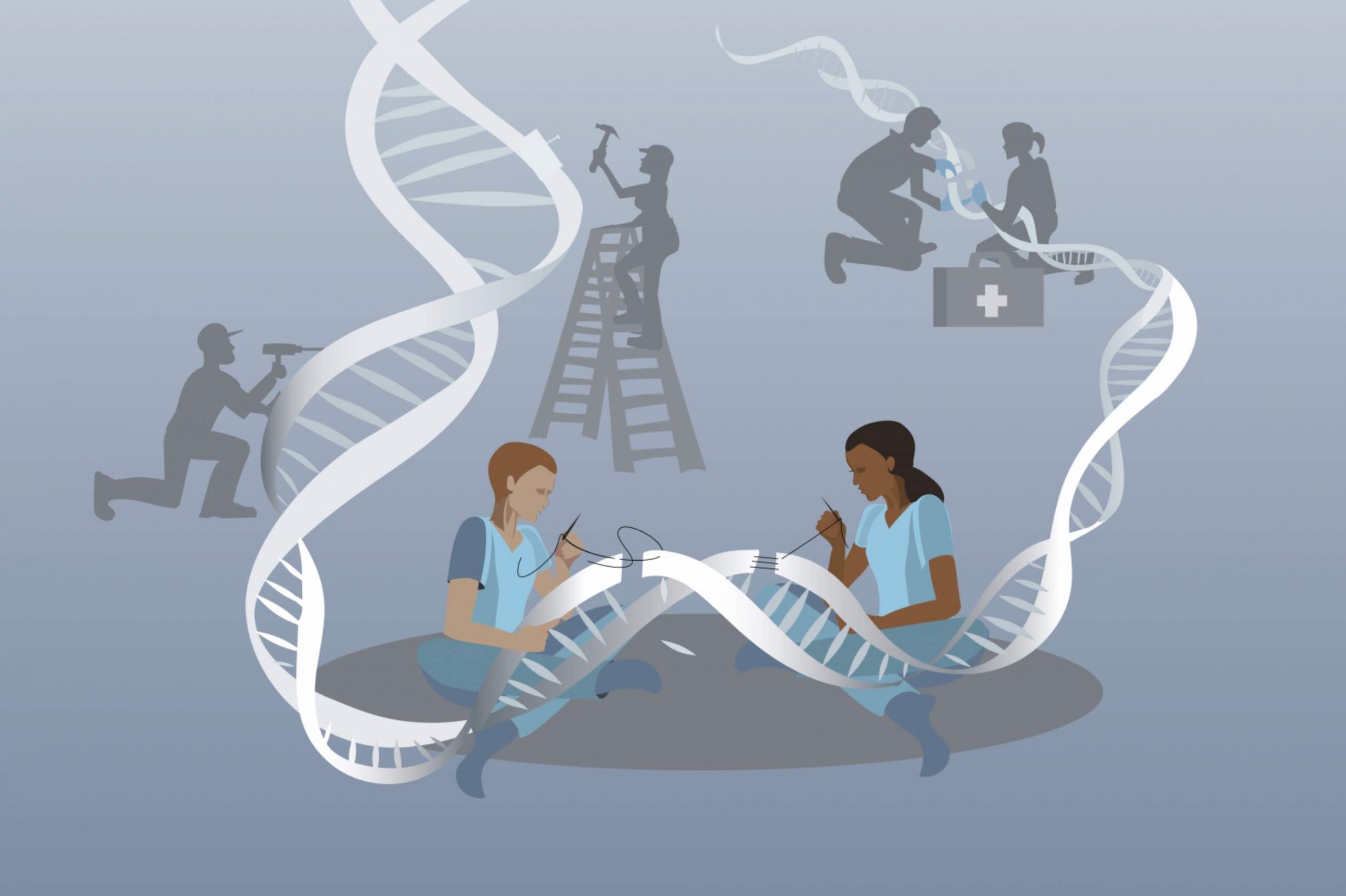
Eva Frederick | Whitehead Institute
October 24, 2021
Gene editing methods often involve breaking a strand of DNA in order to make specific changes to the sequence. They then rely on the cell’s DNA repair pathways to mend the break. These cellular repair pathways, however, are not completely understood, and introduce an element of chance to gene editing; for example, a repair mechanism may patch up the edited strand, but also leave behind an unwanted mutation.
In a paper published online in Cell on October 20, 2021, a collaborative team of researchers in the lab of Whitehead Institute Member, Massachusetts Institute of Technology (MIT) biology professor and Howard Hughes Medical Institute Investigator Jonathan Weissman, the lab of Britt Adamson at Princeton University, and Cecilia Cotta-Ramusino, then a research scientist at Editas Medicine (now at Tessera Therapeutics), present a new experimental method that could help bridge this gap in our understanding.
The method, called Repair-seq, allows researchers to find out which genes and genetic pathways are involved in DNA repair mechanisms. It provides a useful tool for fundamental research on gene repair, as well as a way to test the action of new genome editing methods as they are developed, says Weissman Lab postdoc and first author Jeffrey Hussmann. A companion paper published concurrently in Cell in collaboration with researchers at the Broad Institute of MIT and Harvard provides a glimpse into the utility of Repair-seq when applied to new gene editing technologies.
“The field of gene editing has moved so quickly and people have been so creative developing new methods that our ability to apply them has dramatically outpaced our understanding of exactly how they work,” Hussmann said. “We think that Repair-seq will be a valuable tool going forward so that as new editing methods are developed, we can quickly do a better job of characterizing how they interact with different repair mechanisms.”
Studying repair mechanisms in one fell swoop
Cells have several different methods they use to repair breaks in DNA strands, and the path to any one method depends on a tree of decisions based on the circumstances. “Over decades, a huge number of people have worked out parts of these pathways through focused experiments,” said Adamson, a senior author on both papers and an assistant professor at Princeton University.
Together, the team of researchers saw an opportunity to harness existing CRISPR-based methods to take a broad look at repair pathways in the cell. The method they created combines several CRISPR-based technologies. First, the researchers used a method they previously developed called CRISPRi to inactivate hundreds of genes known to be involved in DNA repair across a cell population. Then, they induced double strand breaks in the cells’ DNA at specific places that the cell would need to heal.
As the cells mended the breaks, the researchers used targeted sequencing to examine the ‘repair outcomes’ — mutations or the lack thereof — in the DNA strand resulting from different methods of repair. Finally, they were able to extrapolate which genes were essential to various repair mechanisms and how they were involved in producing or preventing each type of resulting mutation. They also posted their data online in an interactive format so others can use it to investigate DNA repair genes and pathways.
“This combination of different CRISPR-based technologies has made it possible to, in one fell swoop, recapitulate a lot of the work that was done painstakingly over the past decades to study each repair pathway one at a time,” Hussmann said. “The high-level view of repair that our method produces shows us many of the things that people saw before, and at the same time reveals unexpected connections that we only get by having the comprehensive picture.”
These unforeseen relationships between repair genes may help fundamental researchers refine the decision tree of double strand break repair in the future, said Weissman. “One of the big themes that’s come out of this is that outcomes that superficially look similar can actually have very different mechanisms,” Weissman said.
A ‘prime’ example of Repair-seq’s utility
As the team was developing their Repair-seq methodology, Broad Institute of MIT and Harvard Core Member David Liu’s lab was working on prime editing, a gene editing method that promises more precise control over genetic outcomes than traditional CRISPR methods. Instead of snipping both strands of DNA’s double helix, prime editing makes a ‘nick’ in only one of the strands and introduces a short sequence template containing the desired genetic change.
“When Liu’s group came out with prime editing, our Repair-seq team realized that we had the perfect tool for quickly trying to understand exactly how it was working,” Hussmann said.
The three labs collaborated to use Repair-seq to identify which pathways were at play during the installation of mutations by prime editing, and identified one in particular, called the DNA mismatch repair pathway, that seemed to be interfering with the efficiency and accuracy of the method. When the researchers inhibited this pathway, the performance of Liu’s prime editing technique greatly improved.
“Working with Britt, Jonathan, and their labs has been a beautiful integration of basic science, tool application, and technology development—a real testament to the power of multidisciplinary collaboration” said Liu, also an Investigator of the Howard Hughes Medical Institute.
The researchers also applied Repair-seq to a base editor — a tool to swap specific bases in a DNA sequence — and were able to illuminate the DNA repair genes involved in swapping in particular base.
In the future, the researchers plan to continue adapting the method to new sequencing methods and applying it to new editors as they are developed. “We think Repair-seq is a really practical way of making better genome editors,” Weissman said.
“It has been rewarding to see the efforts of our collaboration come together,” said Adamson. “We hope the insights from our study and tools that those insights have led to will be widely useful to the research community.”
October 22, 2021
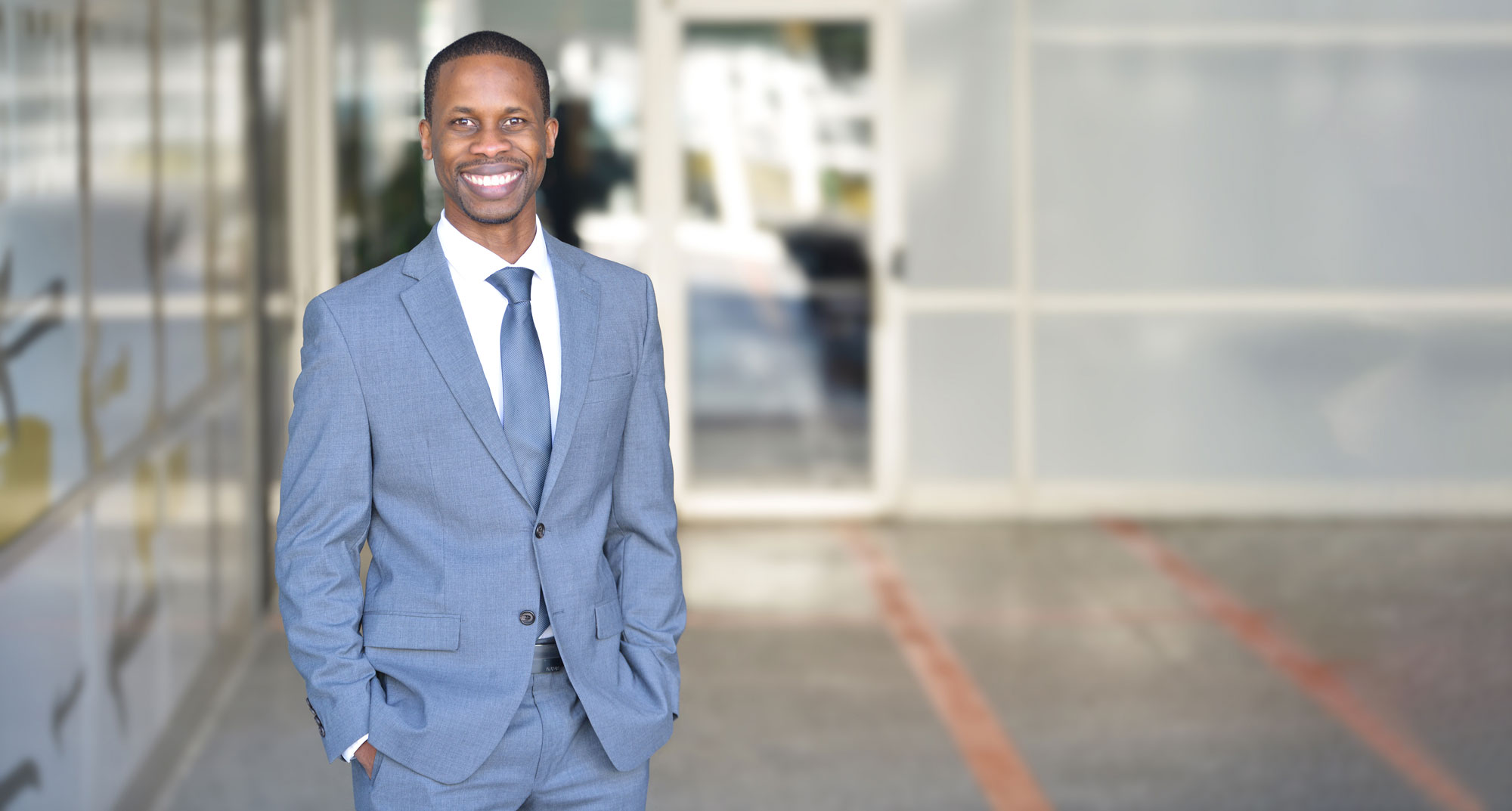
Studying cancer in the Sharp lab helped Courtney JnBaptiste learn strategic thinking skills that he uses as a patent agent, transforming technology into successful biotech businesses.
Raleigh McElvery | Department of Biology
October 17, 2021
Courtney JnBaptiste PhD ’16 spent the first 19 years of his life on the idyllic Caribbean island of Saint Lucia, surrounded by clear waters, sandy beaches, and a robust agricultural community. His family owned their own farm, where they grew bananas and other crops for export. JnBaptiste and his six siblings spent hours each day after school and on the weekends helping to harvest the fruits of their labor. “We were better off than most, but it was a hard existence,” he says. “I had to fight to make something out of life. Where I am today is a big leap.”
He has since moved to the U.S. and completed his PhD at the MIT Department of Biology. Today, he uses the strategic thinking skills he learned during graduate school in his job as a patent agent, helping researchers protect their inventions and start biotech companies.
Despite his exceptional grades, JnBaptiste didn’t enjoy school growing up, and he’d often try to convince his parents that he didn’t need to go to class. His mother, a middle school teacher, was unfazed by his excuses and sent him off to school most days. Despite his protests, JnBaptiste understood his mother’s motto that “education is the key to success.” He knew he’d need good academic standing and self-motivation to attain the financially-stable life he envisioned.
During high school, cable TV became available to his community for the first time, and he was overwhelmed by the deluge of information. He became hooked on Animal Planet and Discovery Channel, and decided that he wanted to be like Jeff Corwin, a biologist, wildlife conservationist, and TV personality. JnBaptiste knew he’d need a college education to reach his career aspirations, and — due to its proximity and promise of opportunity — the U.S. seemed like an ideal destination.
He was accepted to Bethune-Cookman University in Florida and declared a major in biology. He was awarded an environmental research grant, which allowed him to spend several semesters studying the snail populations in Blue Spring State Park. But a summer internship at the University of Kansas Medical Center was what ultimately convinced him to pursue molecular biology rather than environmental sciences. During his junior year, a new biochemistry professor arrived: Christopher Ainsley Davis, a former postdoc in the lab of Cathy Drennan at the MIT Department of Biology.
“When the two of us spoke, he said something that shocked me,” JnBaptiste recalls. “He told me, ‘You’re good enough for MIT and you should apply to their summer research program.’ That just blew my mind. I never thought I was of the MIT caliber — no one had ever challenged me like that before.”
At Davis’ urging, JnBaptiste applied to the MIT Summer Research Program in Biology (MSRP-Bio), and was placed in the lab of Nobel laureate Phil Sharp. In the 1970s, Sharp had co-discovered splicing, a molecular process that happens after DNA has been transcribed into RNA. Segments of the RNA strand are removed, and the remaining parts are stitched back together and translated into proteins that perform vital functions inside the cell.
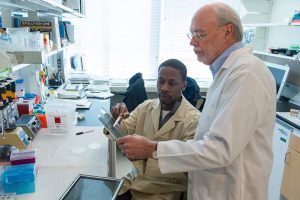
Under the supervision of graduate student and postdoc mentors, JnBaptiste spent the summer of 2009 investigating the role that RNA splicing plays in cancer. “It was the best time of my life,” he says. “I just I loved it. I loved the environment. I loved the lab. I loved MIT. And that experience had a profound impact on me.”
He enjoyed campus so much that he returned just one year later to begin his PhD. He was looking forward to returning to the lab bench, but what he didn’t anticipate was that his first semester of classes would be the most rigorous education he’d ever received. He excelled in biochemistry, but found 7.52 (Graduate Genetics) especially difficult. “It was the first time I’d ever failed an exam,” he recalls.
With the help of mentors, classmates, and tutors, JnBaptiste passed genetics and moved on to the stage of his PhD that he was most excited about: lab rotations. After testing out a few different research groups, he ultimately decided to return to the Sharp lab. In his own words: “It was home.”
JnBaptiste’s thesis project focused on a type of RNA known as microRNA (miRNA), which is never translated into a protein. Instead, it remains in its single-stranded RNA form and helps regulate gene expression. The Sharp lab found that removing all the miRNAs from adult cells prompted dramatic activation of embryonic genes. These genes are typically turned off in adult cells, and only expressed during early development when rapid cell division is required. But they can also be hijacked during cancer to create tumors.
JnBaptiste was surprised to find that adding the miRNAs back into the cells didn’t shut down these embryonic genes. In fact, restoring the miRNAs made the cells divide even more rapidly and increased their ability to form tumors — suggesting “global miRNA restoration” would not be a viable approach to treat cancer.
“This model that we developed showed miRNAs control a very important network in the context of both development and cancer,” JnBaptiste explains. “Cancer occurs when normal cellular processes go awry, so understanding those fundamental molecular interactions is critical to fighting the disease.”
By the time he graduated from MIT in 2016, JnBaptiste knew he enjoyed science, but didn’t have ambitions to run his own lab. Instead, he was curious about how lab experiments and research questions engender companies.
When he was still an MSRP-Bio student, JnBaptiste had met an intellectual property lawyer who’d come to speak on campus. He’d been saving her business card ever since, and reached out to her as his time at MIT was drawing to a close. With her assistance, JnBaptiste was offered a job as a scientific advisor at Goodwin Procter, the international law firm where she worked.
JnBaptiste has since transitioned to a similar role as a patent agent at Pabst Patent Group. There, he collaborates with lawyers to write patents protecting new research technology. While scientists are focused on the minutia of their day-to-day lab experiments, JnBaptiste is tasked with understanding the bigger picture, and how those experiments might lay the foundation for successful businesses that could revolutionize therapeutic approaches.
“As a grad student at MIT, I learned a lot about what it takes to be a strategic thinker in science,” JnBaptiste says. “People like my mentor, Phil Sharp, not only recognize a discovery, they look beyond it to envision its future potential as the next biotech company. That’s a skill I’m still honing as I work to develop my business acumen.”
Looking back at his career trajectory thus far, JnBaptiste is struck by the “beauty and diversity” that comes with earning a degree in biology. “Follow your passions,” he advises, “and surround yourself with people who can see the potential and value in you — even when you cannot yet see it yourself.”