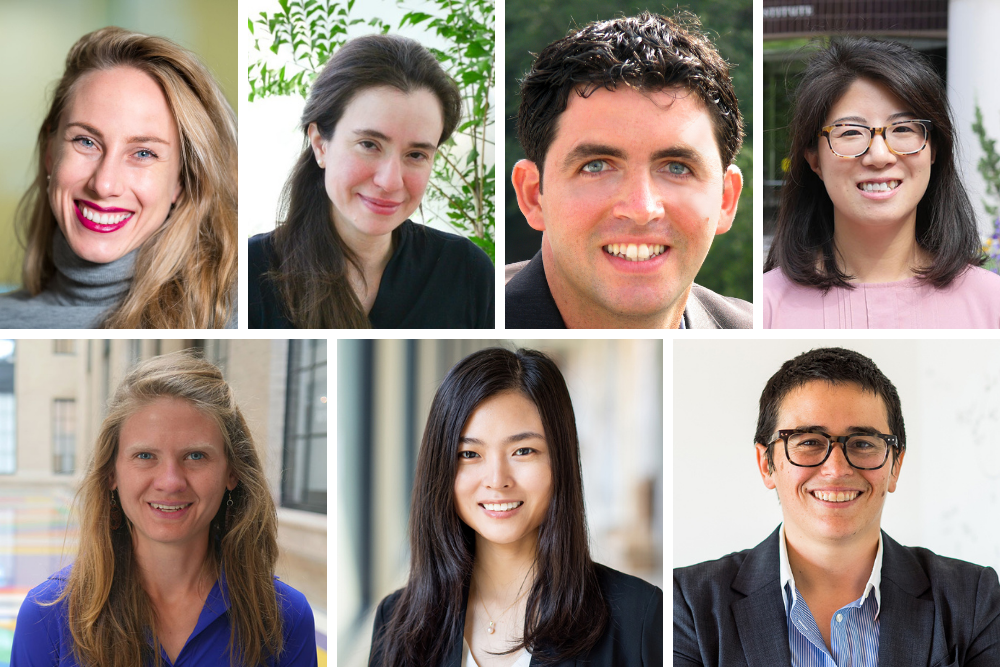
Awards support high-risk, high-reward biomedical and behavioral research.
School of Science
October 8, 2021
On Oct. 5, the National Institutes of Health announced the names of 106 scientists who have been awarded grants through the High-Risk, High-Reward Research program to advance highly innovative biomedical and behavioral research. Seven of the recipients are MIT faculty members.
The High-Risk, High-Reward Research program catalyzes scientific discovery by supporting research proposals that, due to their inherent risk, may struggle in the traditional peer-review process despite their transformative potential. Program applicants are encouraged to pursue trailblazing ideas in any area of research relevant to the NIH’s mission to advance knowledge and enhance health.
“The science put forward by this cohort is exceptionally novel and creative and is sure to push at the boundaries of what is known,” says NIH Director Francis S. Collins. “These visionary investigators come from a wide breadth of career stages and show that groundbreaking science can happen at any career level given the right opportunity.”
New innovators
Four MIT researchers received New Innovator Awards, which recognize “unusually innovative research from early career investigators.” They are:
- Pulin Li is a member at the Whitehead Institute for Biomedical Research and an assistant professor in the Department of Biology. Li combines approaches from synthetic biology, developmental biology, biophysics and systems biology to quantitatively understand the genetic circuits underlying cell-cell communication that creates multicellular behaviors.
- Seychelle Vos, the Robert A. Swanson (1969) Career Development Professor of Life Sciences in the Department of Biology, studies the interplay of gene expression and genome organization. Her work focuses on understanding how large molecular machineries involved in genome organization and gene transcription regulate each others’ function to ultimately determine cell fate and identity.
- Xiao Wang, the Thomas D. and Virginia Cabot Assistant Professor of Chemistry and a member of the Broad Institute of MIT and Harvard, aims to develop high-resolution and highly-multiplexed molecular imaging methods across multiple scales toward understanding the physical and chemical basis of brain wiring and function.
- Alison Wendlandt is a Cecil and Ida Green Career Development Assistant Professor of Chemistry. Wendlandt focuses on the development of selective, catalytic reactions using the tools of organic and organometallic synthesis and physical organic chemistry. Mechanistic study plays a central role in the development of these new transformations.
Transformative researchers
Two MIT researchers have received Transformative Research Awards, which “promote cross-cutting, interdisciplinary approaches that could potentially create or challenge existing paradigms.” The recipients are:
- Manolis Kellis is a professor of computer science at MIT in the area of computational biology, an associate member of the Broad Institute, and a principal investigator with MIT’s Computer Science and Artificial Intelligence Laboratory. He aims to further our understanding of the human genome by computational integration of large-scale functional and comparative genomics datasets.
- Myriam Heiman is the Latham Family Career Development Associate Professor of Neuroscience in the Department of Brain and Cognitive Sciences and an investigator in the Picower Institute for Learning and Memory. Heiman studies the selective vulnerability and pathophysiology seen in two neurodegenerative diseases of the basal ganglia, Huntington’s disease, and Parkinson’s disease.
Together, Heiman, Kellis and colleagues will launch a five-year investigation to pinpoint what may be going wrong in specific brain cells and to help identify new treatment approaches for amyotrophic lateral sclerosis (ALS) and frontotemporal lobar degeneration with motor neuron disease (FTLD/MND). The project will bring together four labs, including Heiman and Kellis’ labs at MIT, to apply innovative techniques ranging from computational, genomic, and epigenomic analyses of cells from a rich sample of central nervous system tissue, to precision genetic engineering of stem cells and animal models.
Pioneering researchers
- Polina Anikeeva received a Pioneer Award, which “challenges investigators at all career levels to pursue new research directions and develop groundbreaking, high-impact approaches to a broad area of biomedical, behavioral, or social science.” Anikeeva is an MIT professor of materials science and engineering, a professor of brain and cognitive sciences, and a McGovern Institute for Brain Research associate investigator. She has established a research program that uniquely combines materials synthesis, device fabrication, neurophysiology, and animal models of behavior. Her group carries out projects that understand, invent, and design materials from the level of atoms to functional devices with applications in fundamental neuroscience.
The program is supported by the NIH Common Fund, which oversees programs that pursue major opportunities and gaps throughout the research enterprise that are of great importance to NIH and require collaboration across the agency to succeed. It issues four awards each year: the Pioneer Award, the New Innovator Award, the Transformative Research Award, and the Early Independence Award.
This year, NIH issued 10 Pioneer awards, 64 New Innovator awards, 19 Transformative Research awards (10 general, four ALS-related, and five Covid-19-related), and 13 Early Independence awards for 2021. Funding for the awards comes from the NIH Common Fund, the National Institute of General Medical Sciences, the National Institute of Mental Health, and the National Institute of Neurological Disorders and Stroke.