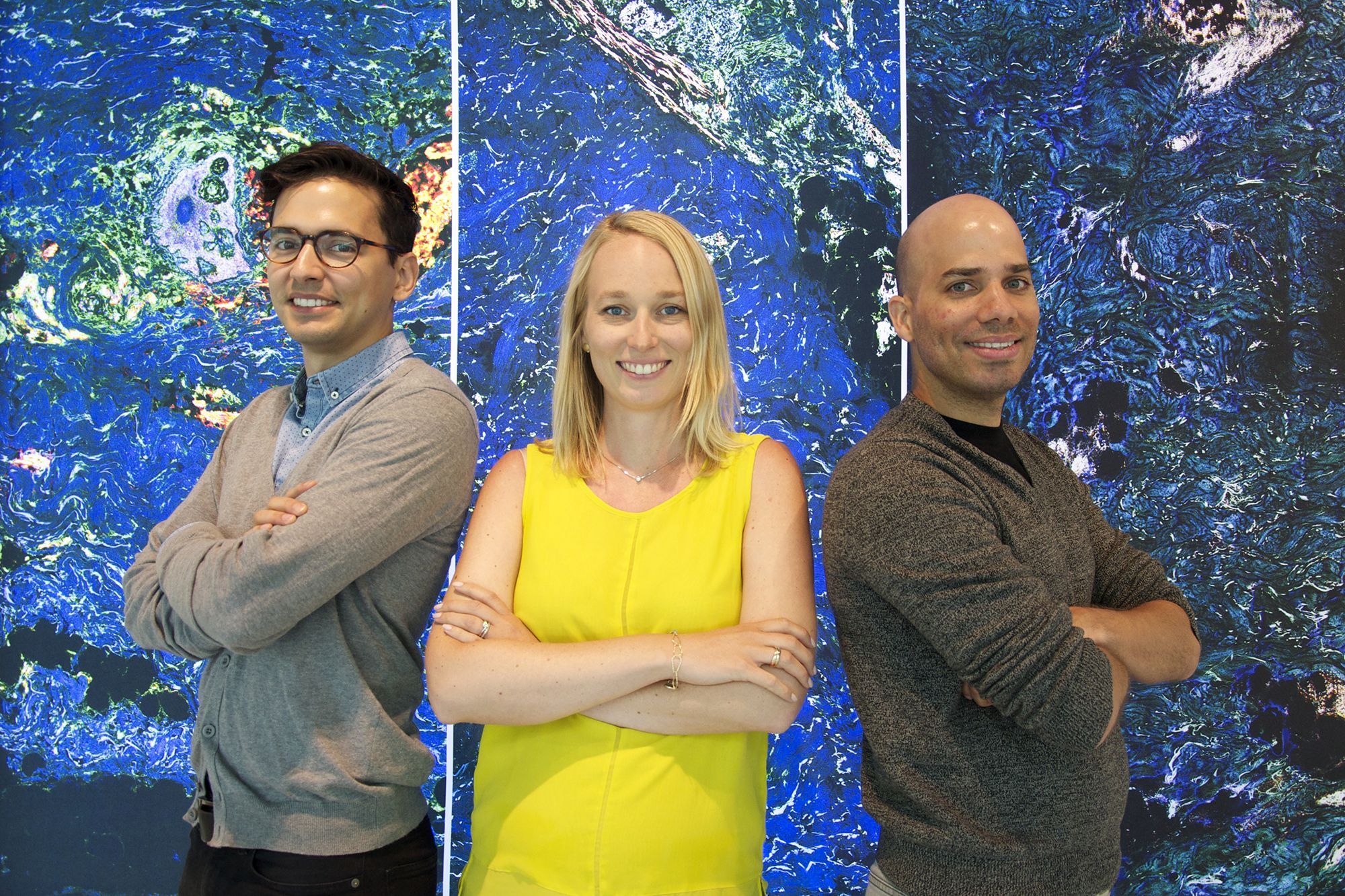
Recent additions bring diverse expertise and cultural perspectives to research community.
Raleigh McElvery | Department of Biology
July 25, 2017
On July 1, MIT Department of Biology welcomed three new faculty members. Since they were all born outside the continental U.S., these newcomers add to the diversity of cultural experiences and contribute to the global face of science at MIT and its affiliated institutions around Kendall Square. The triad also enhances the department’s diverse array of research initiatives. Their interests are as far-reaching as their roots, and range from investigating genetic diseases and cancer immunotherapy to exploiting parasite vulnerabilities.
“When creative individuals with distinct perspectives and approaches come together in an innovative environment like MIT, the possibilities for scientific collaboration and accomplishment are exceptional,” says Alan Grossman, head of the department. “I couldn’t be more pleased to welcome three such outstanding and accomplished individuals into our research community.”
Eliezer Calo
Eliezer Calo is no stranger to MIT. Although he grew up on a farm more than a thousand miles away in the mountains of Carolina, Puerto Rico, Calo first set foot in MIT’s Building 68 11 years ago — and hasn’t wavered in his decision to become a biologist since. In 2006, as part of the MIT Summer Research Program (MSRP), Calo spent 10 weeks studying under Professor Stephen Bell, examining DNA replication. At the time, Calo was a chemistry major at the University of Puerto Rico, but returned post-graduation to MIT’s Department of Biology, earning his PhD while serving as both a teaching assistant for MIT’s course 7.01 (Introduction to Biology) and MSRP program assistant.
“MIT is very unique,” he says. “I’ve done research at multiple institutions, and yet nothing quite compares. Here, the impossible is made possible.”
After completing his postdoctoral training at Stanford University, Calo returned to Cambridge, Massachusetts, this past January as an assistant professor and extramural member at the Koch Institute to head his own lab — exploring the ways in which errors in cellular organelles called ribosomes can lead to disease.
Ribosomes are vital to the translation of genetic code into the molecules integral to life, but are far less often acknowledged for their role in embryonic development. Calo suggests that when ribosomes are not constructed correctly, they are unable to carry out their cellular duties, hindering cell growth and causing developmental disorders. Calo is interested in one condition specifically: Treacher Collins syndrome, which stems from a mutation in a single gene that impedes proper ribosomal assembly. He will soon transfer his experiments from cell cultures to a new model system — zebrafish — in order to further unravel the relationship between ribosome structure and disease.
“The research I do now is purely based on my interest in understanding how cells work,” Calo says. “Specifically, how the mechanisms controlling growth and proliferation operate. These are essential processes that led to the emergence of multicellular organisms, and thus to our own existence.”
Stefani Spranger
One building over in MIT’s Koch Institute, newly-appointed Assistant Professor Stefani Spranger will work to harness the body’s own defense force to pinpoint and eradicate cancer. Spranger carried her passion for immunotherapy across seas from Munich, Germany. As the daughter of two engineers, Spranger was raised on science. “My parents fostered my curiosity,” she says, “which led to my initial motivation to go into science: to figure out how things work.”
While earning her bachelor’s and master’s degrees from the Ludwig-Maximilians University of Munich, Spranger discussed publications focusing on two clinical trials that used engineered immune cells to combat malignant melanoma. These publications ignited Spranger’s enthusiasm for immune-based therapies, which in turn spurred her doctoral and postdoctoral training at the Helmholtz-Zentrum Munich in the Institute for Molecular Immunology and the University of Chicago, respectively.
While Spranger’s education helped hone her immunology research skills, she is excited to experience a more varied academic environment encompassing a range of disciplines. “I was drawn to MIT because of its diverse faculty and the breadth of research interests,” she says.
Spranger’s lab will employ tumor models in mice to determine how cancer and immune cells interact. In particular, she aims to discern the many factors related to the cells, tissues, and environment that could affect the immune system’s anti-tumor response. Ultimately, Spranger hopes to contribute to new treatments that trigger the body’s defense to thwart cancer.
Sebastian Lourido
Trained as both an artist and a scientist, Sebastian Lourido works to counter an entirely different kind of invader spreading biological mayhem: parasites. Originally from Colombia, he was recently named assistant professor of biology, joining the cohort of 15 faculty members at the Whitehead Institute for Biomedical Research — one of just 28 individuals to ever receive this appointment. The title may be new, but this is familiar turf for Lourido. He became a Whitehead Fellow in 2012, after receiving his PhD in microbiology from Washington University in St. Louis and a bachelor’s in studio art and cell and molecular biology from Tulane University. A pioneer in more ways than one, Lourido formed his own lab as a fellow rather than following a more conventional postdoc path.
Lourido spent much of his childhood exploring his mother’s genetics lab, where he analyzed practically anything he could fit under a microscope. “That experience solidified my excitement for the invisible mechanisms that make up the living world,” he says. “I can’t remember a time when I didn’t know that genetic information was carried in our cells in the form of DNA, and passed from one generation to the next.”
Constantly seeking ways to merge his artistic endeavors with scientific ones, Lourido leverages his creativity to glean insight into the systems and structures that constitute life. He probes a group of microscopic invaders known as Apicomplexan parasites, revealing their weaknesses in order to devise potential treatments. Lourido’s team was the first to perform a genome-wide functional analysis of an apicomplexan — gaining deeper understanding of the genes and molecules key for the invasion process. In 2013, Lourido received the National Institutes of Health (NIH) Director’s Early Independence Award, and with it a five-year grant to investigate motility in one kind of parasite, Toxoplasma gondii. This same interloper is also the subject of Lourido’s two-year NIH-funded project, for which he is the principal investigator.
Lourido has found the MIT community both welcoming and inclusive. Even as he was interviewing for his new position, he was struck by the collaborative, collegial, and nurturing environment.
“This level of engagement permeates the other elements of our community — students, postdocs, and staff scientists — who drive the exciting research happening every day at MIT,” he says. “There are many forces that shape the diversity of our campus, and we need to be vigilant and work hard to continue to encourage and support scientists from different backgrounds, experiences, and cultures.”