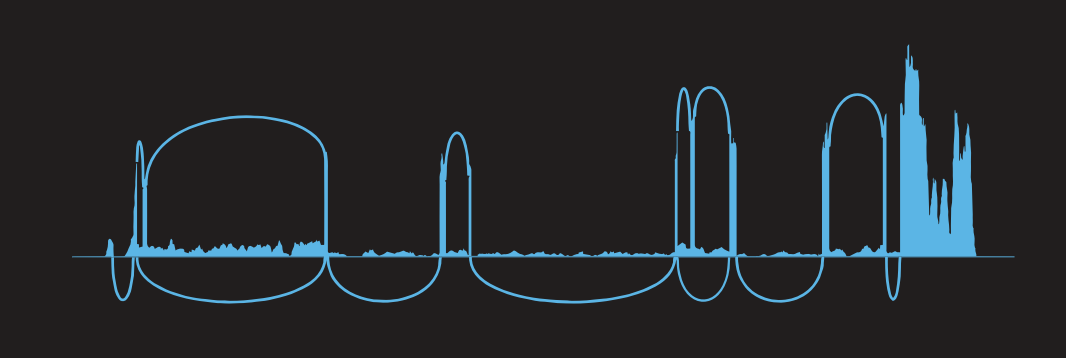
How do cells respond to disruptions in splicing?
Lillian Eden | Department of Biology
March 4, 2024
New research from the Calo Lab in the Department of Biology has identified the protein Mdm2 generating a form that activates a cascade of cellular stress responses when splicing is disrupted.
To create proteins, DNA is transcribed into RNA, and that RNA is then “translated” into protein. Between the creation of the RNA and the translation to protein is often a step called splicing. During splicing, segments called introns are removed, and the remaining pieces, called exons, are joined together to form the blueprint for translation. By splicing together different exons, the cell can create different proteins from the same section of genetic code. When splicing goes awry, it can lead to diseases and cancers.
New research recently published in Disease Models & Mechanisms from the Calo Lab in the Department of Biology at MIT has identified the mechanism for how cells respond to disruptions in splicing, which involves activating a cellular stress response. The stress response, once activated, causes widespread effects, including changes to cell metabolism.
Researchers have discovered cellular stress responses for other core cellular processes, such as ribosome biogenesis. However, this is the first time researchers have identified how cells respond to perturbing the splicing process.
A particular protein acts as a kind of canary in a coal mine: Mdm2, which responds to a broad range of splicing disruptions. Mdm2 does not cause a stress response by itself. Rather, Mdm2 is itself spliced differently in response to splicing disruptions. Downstream, the alternative splicing of Mdm2 leads to the activation of a protein called p53, which is known to orchestrate a cascade of responses to stress.
Researchers have long wondered why some cell types seem more sensitive to splicing disruptions than others. For example, some disorders caused by mutations in proteins that perform RNA splicing, despite affecting the whole organism, induce more noticeable changes in tissues derived from the neural crest—a collection of stem cells that contributes to the formation of the face, jaw, retinas, limbs, and heart during development. Certain splicing inhibitors have also increased the effectiveness of some cancer treatments, but the mechanism is unknown.
One of the p53-induced stress responses includes changing the metabolism of cells and how they use sugars, which may explain why some cells are more sensitive to splicing disruptions than others. Inhibiting glycolysis, the reactions that extract energy from glucose, can affect how cells divide and migrate.
The way cells divide and migrate is critical during development; in experiments, zebrafish treated with glycolysis inhibitors exhibited similar changes to craniofacial features as those where splicing was disrupted. Cancerous cells, too, are known to require high levels of sugar metabolism and, therefore, may be especially sensitive to treatments that induce changes in the splicing pathway.
The researchers knocked down genes to mimic milder splicing disruptions instead of knocking them out entirely. Splicing is so essential that knocking out the splicing machinery can lead to extreme responses like cell death. In organismal models like zebrafish, those severe phenotypes don’t accurately reflect how splicing disruptions present in human diseases.
First author Jade Varineau, a graduate student in the Calo lab, was drawn to the project because it allowed her to explore what was happening at the RNA and cellular level while also observing how splicing perturbations were affecting the whole organism.
“I think this data can help us reframe the way we think about diseases and cancers that are impacted by splicing—that a treatment that works for one may work for another because all the symptoms may stem from the same cellular response,” Varineau says.
Although the results indicate how cells broadly respond to splicing perturbations, the mechanism for how disruptions in splicing induce the alternate splicing of Mdm2 remains unclear. Senior author Eliezer Calo says the lab is also exploring how splicing mechanisms may be altered for things like cancer. Their work, he says, opens the door for further exploration of cell-type specificity of genetic disorders and improvements in cancer treatments using splicing inhibitors.
“We know that the sensor is encoded in the gene Mdm2—what are the molecules that allow Mdm2 to act as a sensor, and how does the sensor malfunction for things like cancer?” Calo says. “The next step is to find out how the sensor works.”