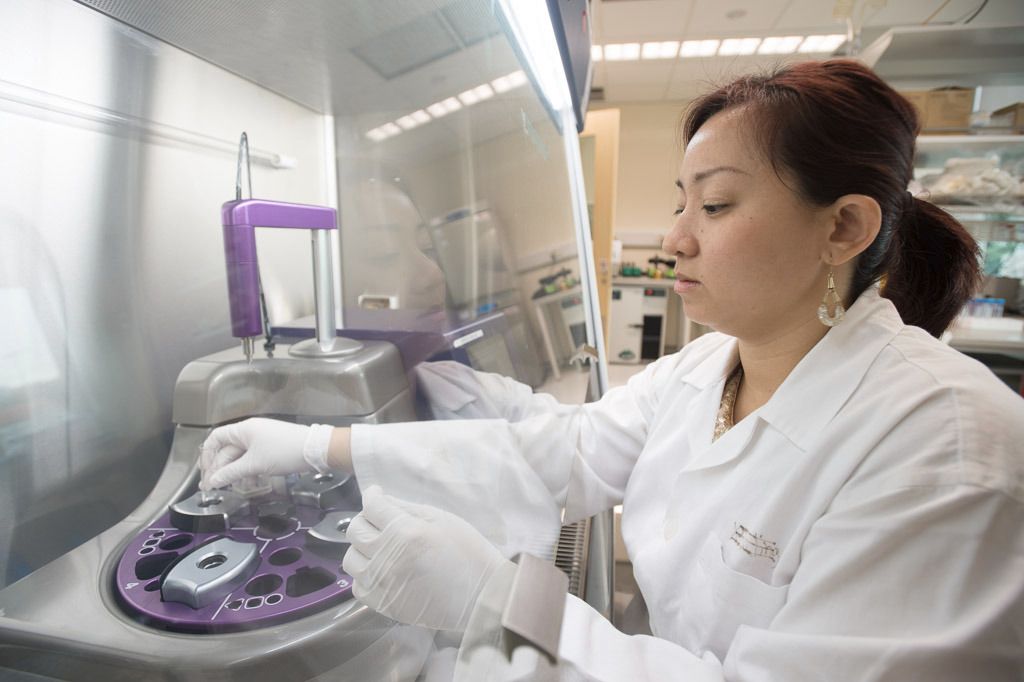
Singapore-MIT Alliance for Research and Technology
August 13, 2020
Researchers from the Singapore-MIT Alliance for Research and Technology (SMART), MIT’s research enterprise in Singapore, have found a practical way to induce a strong and broad immunity to the dengue virus based on proof-of-concept studies in mice. Dengue is a mosquito-borne viral disease with an estimated 100 million symptomatic infections every year. It is endemic in over 100 countries in the world, from the United States to Africa and wide swathes of Asia. In Singapore, over 1,700 dengue new cases were reported recently.
The study is reported in a paper titled “Sequential immunization induces strong and broad immunity against all four dengue virus serotypes,” published in NPJ Vaccines. It is jointly published by SMART researchers Jue Hou, Shubham Shrivastava, Hooi Linn Loo, Lan Hiong Wong, Eng Eong Ooi, and Jianzhu Chen from SMART’s Infectious Diseases and Antimicrobial Resistance (AMR) interdisciplinary research groups (IRGs).
The dengue virus (DENV) consists of four antigenically distinct serotypes and there is no lasting immunity following infection with any of the DENV serotypes, meaning someone can be infected again by any of the remaining three variants of DENVs.
Today, Dengvaxia is the only vaccine available to combat dengue. It consists of four variant dengue antigens, one for each of the four serotypes of dengue, expressed from attenuated yellow-fever virus. The current three doses of immunization with the tetravalent vaccine induce only suboptimal protection against DENV1 and DENV2. Furthermore, in people who have not been infected by dengue, the vaccine induces a more severe dengue infection in the future. Therefore, in most of the world, the vaccination is only given to those who have been previously infected.
To help overcome these issues, SMART researchers tested on mice whether sequential immunization (or one serotype per dose) induces stronger and broader immunity against four DENV serotypes than tetravalent-formulated immunization — and found that sequential immunization induced significantly higher levels of virus-specific T cell responses than tetravalent immunization. Moreover, sequential immunization induced higher levels of neutralizing antibodies to all four DENV serotypes than tetravalent vaccination.
“The principle of sequential immunization generally aligns with the reality for individuals living in dengue-endemic areas, whose immune responses may become protective after multiple heterotypic exposures,” says Professor Eng Eong Ooi, SMART AMR principal investigator and senior author of the study. “We were able to find a similar effect based on the use of sequential immunization, which will pave the way for a safe and effective use of the vaccine and to combat the virus.”
Upon these promising results, the investigators will aim to test the sequential immunization in humans in the near future.
The work was supported by the National Research Foundation (NRF) Singapore through the SMART Infectious Disease Research Program and AMR IRG. SMART was established by MIT in partnership with the NRF Singapore in 2007. SMART is the first entity in the Campus for Research Excellence and Technological Enterprise (CREATE) developed by NRF. SMART serves as an intellectual and innovation hub for research interactions between MIT and Singapore, performing cutting-edge research of interest to both Singapore and MIT. SMART currently comprises an Innovation Centre and five IRGs: AMR, Critical Analytics for Manufacturing Personalized-Medicine, Disruptive and Sustainable Technologies for Agricultural Precision, Future Urban Mobility, and Low Energy Electronic Systems. SMART research is funded by the NRF Singapore under the CREATE program.
The AMR IRG is a translational research and entrepreneurship program that tackles the growing threat of antimicrobial resistance. By leveraging talent and convergent technologies across Singapore and MIT, they aim to tackle AMR head-on by developing multiple innovative and disruptive approaches to identify, respond to, and treat drug-resistant microbial infections. Through strong scientific and clinical collaborations, they provide transformative, holistic solutions for Singapore and the world.