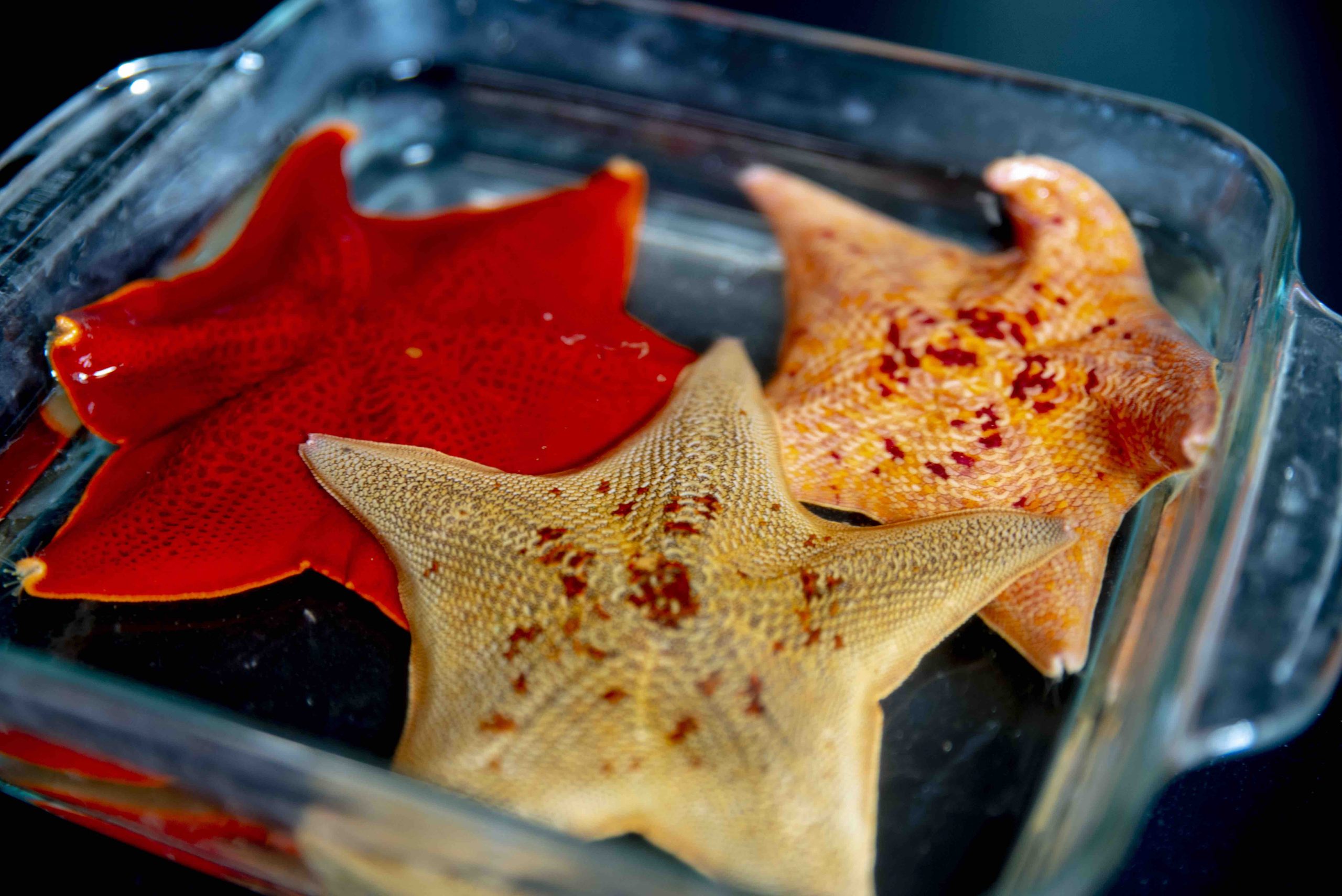
Greta Friar | Whitehead Institute
February 25, 2020
Meet the stars
Zak Swartz, a postdoctoral researcher in Whitehead Institute Member Iain Cheeseman’s lab, gets an unusual delivery a few times a year. It comes in a cardboard box a few feet in length on each side with a “perishable” label on top. When the most recent box arrived, Swartz took it to a small, chilly room across from Cheeseman’s lab. He cut and tore his way through several layers of packaging, insulation, and cold packs to get to his prize: a bunch of plastic bags half-filled with water, the sort of thing that might contain a goldfish at a county fair. Instead of goldfish, these makeshift aquaria each contained two or three bat stars (Patiria miniata), a hardy species of starfish seemingly unphased by their transcontinental trip in a cardboard box.
Bat stars are so named because the thick webbing between their arms—of which they typically have five, though they can have up to nine—gives their short limbs a bat-wing-like appearance. The stars are most often some shade of red or orange, but come in a variety of colors and patterns. Each star that Swartz pulled out of the box had a unique design coating its body.
Swartz untied the plastic bags and took the bat stars out one by one. He gently dropped each star into an aquarium in the corner of the room, where it would sink slowly to the bottom, then crawl to the sides and inch its way up, clinging to the glass. Although their movements are barely perceptible to a human watching, within minutes bat stars coated the walls of the aquarium, the tiny tube feet on the underside of each arm sticking them firmly in place.
As the first round of bat stars settled onto their chosen perches, Swartz returned to the box. He took another bat star out of its bag and held it in his hand for a moment.
“This one’s heavy,” he said, with satisfaction; heavy stars are more likely to be full of eggs, and that’s what Swartz is interested in. He’s researching how cells, such as immature egg cells, that remain dormant or non-dividing for a long time retain their ability to divide. The proteins necessary for cell division degrade over time, like parts of a machine rusting and breaking down, and yet many cells remain able to divide long after their unused cellular machinery should have become useless. In the case of humans, precursor egg cells can spring back into action after decades of dormancy in the ovaries, and can go on to perform that most impressive feat of cell division: the creation of a whole new organism from one cell. But human eggs are not the most accessible or readily available research material, and so Swartz has turned to the bat stars, an excellent source of reproductive cells, to help answer his questions.
Fertile ground for discovery
Bats stars reproduce by spawning. The females release millions of eggs into the ocean through pores in between their arms, while at the same time males release clouds of sperm. The reproductive characteristics of bat stars make them ideal research animals for Swartz. They have a long breeding season during which they can ovulate, they produce millions of eggs at a time, and they release these eggs out into their environment, where they develop externally.
In the lab, Swartz must extract the immature egg cells before they are released, so he can study the processes that take place in the cells during their development. This is much easier than extracting cells from mammalian ovaries; all it requires is a minimally invasive procedure from which the starfish quickly recover. Once Swartz has extracted the eggs in their “hibernating” pre-spawn state, he can control and observe all of the steps of their development, from their re-activation through to fertilization and beyond.
One reason Swartz has chosen bat stars over other starfish species is because of their hardiness. Bat stars fare well in a laboratory aquarium—they even do well being shipped in a cardboard box—whereas other species that Swartz considered using are less adaptable to these conditions. So, while it may have been more convenient to use a species from local Atlantic waters, in the interest of maintaining a healthy lab population of specimens, Swartz has had to procure his research subjects long distance. The geographical range of bat stars is the stretch of Pacific Ocean along the coast of North America.
How are the animals getting from the Pacific coast to Whitehead Institute? They are collected by a contact Swartz made several years ago in California.
Scuba diving for science
Josh Ross runs a research specimen procurement company based in San Pedro, California called South Coast Bio-Marine. Ross has been collecting starfish for Swartz since 2015, and he also collects a variety of marine animals, from sea urchins to limpets to nudibranchs, for researchers at other institutions. Specimens from South Coast Bio-Marine have been used in research on, among other topics, fertilization, memory formation, sleep, and shape changes in oocytes.
Most mornings, Ross and his employees load up a boat with scuba gear and equipment and head to their chosen dive spots, where they collect specimens for the first half of the day.
“I love the fact that I get to dive and work in the ocean every day. When Monday comes, all the weekend boaters and fishermen go back to their jobs, and we have the ocean almost all to ourselves. We are out in the wilderness with truly wild ocean creatures,” Ross says—though sometimes those wild creatures can interfere with their collection plans.
The team then take what they have collected back to Ross’ lab, where the specimens are kept in chilled seawater tanks for a few days to acclimate before Ross ships them to researchers. Ross takes care to harvest specimens with sustainability in mind. When finding starfish for Swartz, he will only take animals that have had time to grow large and spawn several times in their native habitat before being collected.
Ross searches for bat stars at dive sites in 55- to 70-foot deep water, either out in the open, on rocky shelf reefs, or on the sand near the reefs. Bat stars’ habitat ranges from the low intertidal zone, the part of the seashore that’s covered with water except at low tide, into the mild depths of the subtidal zone. They live among kelp and surf grass forest, and use the numerous tube feet lining the underside of each arm to crawl across the sandy ocean floor and cling to rocks. Instead of blood, starfish pump sea water through a water vascular system to circulate nutrients through their bodies and control their limbs. They pump water into and out of their tube feet to make them extend and contract, allowing the stars to move. The tube feet can also release glue-like chemicals that help the stars adhere to rocks even in the strong currents of the ocean—or cling to glass walls in aquariums.
Bat stars are voracious eaters—scavengers as well as predators—and Ross often finds them in the middle of a meal. Bat stars eat by extending their stomachs out of their bodies to dissolve their prey in digestive juices, then drink it up. This system allows them to eat larger prey than their small mouths would otherwise allow. A second stomach that remains inside the star further digests the food.
Ross tries to select starfish for Swartz that feel “ripe,” meaning they are ready or nearly ready to spawn. Indicators that Ross uses include ripe stars having larger “shoulders” and being puffier than unripe stars. After Ross has collected enough bat stars, he ships them from California to Cambridge, Massachusetts, where their role in Swartz’ research begins. Swartz is interested in female specimens, but there is no good way to identify a starfish’s sex on sight, so the bat stars that Ross sends to Swartz tend to be fifty-fifty female and male. Swartz must examine a small biopsy of the gonad to find out which of them contain oocytes, the immediate precursor cells to fertilizable eggs. Once identified, the animals are separated into two aquariums by sex, ready to provide oocytes for experiments. Swartz feeds the starfish a steady diet primarily consisting of raw, peeled shrimp, which keeps them developing new oocytes. When the starfish have completed their time in the lab, Swartz tries to donate them to local aquariums.
Eggs with answers: What we’ve learned from bat stars
Swartz is using the bat stars to investigate how cells divide—specifically, how cells retain the ability to divide after long periods without doing so, and how cell division processes are adapted to the context of animal reproduction and development. Whitehead Member Iain Cheeseman’s lab, where Swartz is a postdoc, investigates the cellular machinery required for cell division. In particular, Cheeseman’s team studies the kinetochore, a complex of proteins involved in orchestrating the precise segregation of chromosomes during cell division, and the centromere, the region in the middle of the chromosome where the kinetochore assembles. The centromere is not defined by its DNA sequence, but by proteins that attach there and signal the kinetochore to assemble at that location. One of the necessary proteins that marks the centromere is called CENP-A. Without CENP-A, the centromere won’t function properly, so chromosomes won’t be correctly distributed into the two new cells created during cell division. However, as with other proteins, there was an open question whether CENP-A degrades over time. Once it is lost at the centromere the cell cannot get it back, and loses the ability to divide. This fact caused Cheeseman and Swartz to wonder how cells that spend long periods of time without dividing can start up again. What sort of maintenance do cells need to do to keep their cell division machinery operational?
Eggs and oocytes, the precursor cells that will develop into eggs, are a great test case because they remain non-dividing for a very long time. Swartz harvested the bat stars’ oocytes and used a fluorescent tag to track the quantity of CENP-A inside of the cells as they progressed through their cell cycle stages. To get a close look at what happens to the CENP-A in oocytes during their dormancy, Swartz maintained the cells in a state of arrested development in petri dishes by putting them in a mixture he calls “starfish juice,” a blend of culture fluids, antibiotics, and some of the bat stars’ own natural fluids.
With the help of the fecund bat stars, Swartz and Cheeseman found the answer to their questions about CENP-A. In research published in Developmental Cell in 2019 [4], the scientists discovered that cells slowly replace their CENP-A over time, swapping out the old protein at risk of breaking down with new functional protein. This finding upended the previous understanding of CENP-A as a static protein that was placed on the centromere once and then remained as long as it could. The researchers also tested a human cell line that can enter dormancy and divide later, and found that, like the sea star oocytes, those cells gradually exchanged CENP-A. In contrast, the researchers discovered that cell types that never need to divide again, like muscle cells or other specialized cells, let most of their CENP-A degrade and so permanently lose the capacity to divide. This finding means that the presence of CENP-A may be a good indicator for use in determining whether any given cell retains the ability to divide in the future. The question of a specialized, or terminally differentiated, cell’s potential for renewed cell division is of great interest in regenerative medicine research. Indeed, this work sparked collaboration between Cheeseman, Swartz and Whitehead Institute Fellow Kristin Knouse, who studies regeneration in mouse and human cells.
The findings could also explain why tissues like muscle rarely develop cancers; the cells cannot replicate and so cannot grow tumors. Furthermore, Swartz thinks that their findings could prove valuable for assisted fertility research.
“Understanding the natural biology that keeps eggs in good shape, able to resume and finish their development after long dormancies, could provide insight into what goes wrong when eggs do not remain viable,” Swartz says.
The possibilities for future research spawning from Swartz’ work are many. The advances that may come, whether in regenerative medicine, assisted fertility, or elsewhere, will all be owed in part to a group of bat stars that travelled across a continent, from ocean to ocean, in a chilled cardboard box to help unravel the mysteries of cell division.