Our advanced undergraduate seminar courses focus on the primary research literature, with the goal of introducing you to the methods of contemporary biological research and the logic of experimental design and interpretation. The seminars are taught by postdoctoral scientists who are practicing researchers. They will expose you to the kind of thinking that is central to contemporary biological research and also impart specific knowledge in particular areas of biology.
Advanced seminars are graded pass/fail, carry six units, and meets for two hours weekly.
Advanced seminars feature:
- Small class size (limit of 8 students)
- High degree of personal contact with the instructor
- Focus on the primary research literature
- Lively discussions about biological problems
Prerequisites:
7.06 Cell Biology or 7.28 Molecular Biology
Subject offering descriptions
Days and class times are flexible and will be determined at the first class meeting.
Fall 2023
7.341 Engineered Organs: Design & Fabrication for Use in Research & Medicine
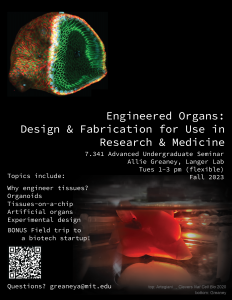
Instructor: Allie Greaney (laboratory of Robert Langer)
Transplanted organs will someday be custom engineered specifically for each patient from their own cells. Today researchers commonly test new drugs and other treatments using engineered tissues in bioreactors instead of animals. In academic laboratories, engineered tissue models allow researchers to ask and answer biologic questions that would be impossible to test in humans. These advances have been made possible by leveraging various scientific and engineering principles to build tissues and organs from scratch outside the body. In this course students will learn how engineered tissues and organs are revolutionizing research and medicine through reading and discussing the primary research literature. We will cover the fundamentals of how to design, fabricate, and utilize engineered tissues in biological research, in pharmaceutical testing, and in the clinic. Topics will include organoids (clusters of cells grown in 3D culture), tissues-on-a-chip, and artificial whole organs. We will compare and contrast fabrication methods and design criteria across organ systems. For example, how do we make heart tissues beat in a dish, or lung tissues breathe outside the body? How important are these factors in generating a tissue with the needed properties? How do we assess if an organ is ready to be transplanted? All readings, discussions, and activities will be centered around conceptually teaching students how to design experiments well and analyze outcomes efficiently, accurately, and critically. To emphasize relevant skills, the major written assessment will be a Specific Aims page (overview of a grant proposal) proposing a project that leverages engineered tissues. As a final project, students will orally present their own analysis of a research article about engineered tissues. This course will include a field trip to the Cambridge biotechnology company Vivtex to learn about the use of engineered tissues in industrial research. Any student interested in this topic is welcome, including but not limited to those interested in careers in biomedical research and/or medicine. By the end of the course, students should be equipped with the basic knowledge and critical thinking skills necessary to conduct and interact with research in the field of tissue and organ engineering.
7.342 Decoding Cellular Complexity: Modeling Networks for Biological Insight
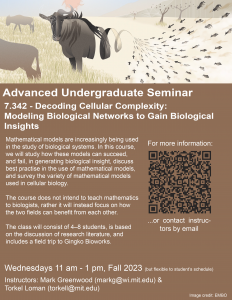
Instructors: Mark Greenwood (laboratory of Pulin Li) and Torkel Loman (laboratory of Alan Edelman)
Cells exist in extraordinarily complex environments; hundreds, if not thousands, of signals fluctuate within and outside of a cell. Nonetheless, cells have abilities to make sense of this complexity, and appropriately interpreting and responding to these signals is crucial to a myriad of biological decisions, such as life v. death, flowering v. growth, and male v. female, to cite just a few examples. How do cells accomplish this remarkable feat? In this course we will focus on two questions: (1) How do cells use transcriptional networks to process cellular signals? and (2) As researchers, how can we combine experiments and mathematics to make sense of transcriptional networks? A principal objective of this course is to showcase the practical utility of mathematical modeling in biological research. The course should be of interest both to biologists interested in understanding how models are applied in biology research and to mathematicians interested in applying modeling to biological research. We will discuss how various cellular models work, in what contexts they can be useful, and in what contexts they are not. We will consider the different scales at which transcriptional networks can be abstracted, from three gene interactions to metabolic networks with hundreds of genes. We will focus on the primary research literature, discussing how network features provide signal processing capabilities that enable cells to achieve important biological functions. Examples will be drawn from across biology, including T cell differentiation in the immune system, plant circadian clocks, and the bacteria stress response. In each case, we will emphasize how mathematical methods enabled researchers to solve a problem. Students will have the opportunity to simulate mathematical models and apply them to ask biological questions. We will visit the biotechnology company Ginkgo Bioworks, where network modeling is used to engineer cells for real world applications, such as digesting ammonia in patients with metabolic disease, and producing enzymes critical for the vaccine supply chain. The course will conclude with a short oral critique by each student on a primary research paper relevant to the course but not discussed in class. By the end of this course, we hope students will gain the ability to understand and critique mathematical studies of biology and an appreciation of the value of this approach for exploring complex biological problems.
7.343 Drug Delivery for Human Disease: Overcoming the Body’s Barriers to Develop New Therapies
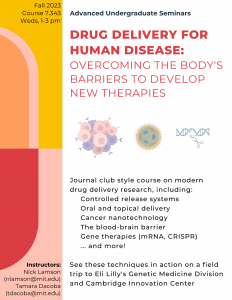
Instructors: Nick Lamson and Tamara Dacoba (laboratory of Paula Hammond)
The human body has developed biological barriers to efficiently exclude foreign entities from entry. While an important defense against infection and disease, these barriers also impede the delivery of therapeutic drugs to their target organs and tissues. This course will explore a wide breadth of primary research articles examining the body’s barrier tissues, the metabolic processes that affect drug efficacy, and current drives to develop new strategies for drug delivery and efficacy. During this literature-based course, we will discuss methods of measuring pharmacokinetics (the absorption, distribution, metabolism, and secretion – ADME – of drugs within the body) and strategies to improve their pharmacokinetic properties using polymeric controlled-release materials to increase solubility and stability. We will discuss the gastrointestinal tract as a series of barriers to oral administration of drugs – including small molecules, peptides and proteins, and nucleic acids – and drug-delivery materials that aim to improve oral drug bioavailability. We will study the use of chemical and physical disruptors of the skin to bypass this barrier. We will explore nanoparticle design based on the encapsulated drugs, or to leverage the unique biological environment in the context of cancer. Finally, we will discuss the challenges facing the new wave of nucleic acid and gene therapies – including siRNA, mRNA, DNA, and CRISPR technologies – to treat diseases throughout the body, as well as the nanoscale drug-delivery carriers that promise to enable such approaches. Finally, we will examine the blood-brain barrier (BBB) as a formidable gatekeeper against drug delivery for brain cancers and neurological diseases, and strategies to overcome the BBB via molecular design, drug carrier development, or avoidance of the barrier altogether. To complement our discussions of articles from the primary research literature, we will participate in a field trip to Eli Lilly’s Cambridge Innovation Hub, in the nearby Kendall Square biotech hub. There, we will discuss ongoing drug delivery research and potential career paths with members of the Genetic Medicine division, connecting their scientific efforts and future research outlook with the principles discussed in earlier meetings of the class. Overall, this course will provide training in reading, analyzing, and critiquing cutting-edge scientific studies, culminating in each student delivering a “Journal Club” style presentation about a relevant research article. This class should be particularly beneficial to students interested in careers in biomedical research, pharmaceutical development, and/or medicine, but we welcome all students who would like to learn to critically evaluate primary scientific literature, understand scientific reasoning and experimental design, and learn about ongoing areas of development of new therapeutic strategies.
7.344 Mortal Beings, Immortal Cells: Cellular Immortality in Normal Biology and Human Disease
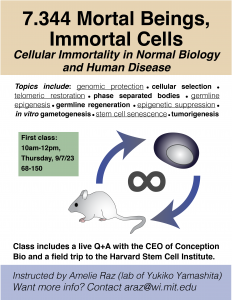
Instructors: Amelie Raz (laboratory of Yukiko Yamashita)
All life relies on cellular immortality: although living individuals are mortal, genomes have been passed down with remarkable fidelity through immortal cell lines for as long as life has existed. Every cell in every human body arose from two germ cells, and each of these germ cells was produced from a body that itself arose from two germ cells, and so on since the origin of cellular life. Cellular immortality, though, has a dark side: uncontrolled cell survival is a driving force of cancer. In this class, we will examine cutting-edge data concerning the features of cellular immortality, how cellular immortality functions in normal biological systems, how cellular immortality can be induced in otherwise mortal cells, and what happens when cellular immortality goes awry. First, we will discuss how cellular immortality requires (1) strict genomic protection against damaging agents; (2) cellular competition and selection for the most intact genomes and organelles; and (3) regeneration of lost components. Next, we will examine how germline immortality is inherited from generation to generation, with a special focus on the nature and necessity of intracellular phase-separated bodies known as “germ granules.” We will discuss the frequent use of inductive signals to determine the germline, including for mammalian immortal cells, as well as the molecular networks required to induce the germline fate in vivo. One active area of current research concerning inductive germline signaling involves methods for inducing the germline fate ex vivo, and we will discuss how to engineer immortality in a dish. Finally, we will examine cellular immortality in a pathogenic context and discuss how cancer cells co-opt natural developmental inducers of immortality with consequences that are detrimental to the organism. This course will include a Q+A session with the CEO of Conception Bio, a startup focused on generating ex vivo oocytes with the features of natural cellular immortality, and an in-person field trip to the laboratory of Professor Ya-Chieh Hsu of the Harvard Stem Cell Institute to learn about approaches to studying stem cell aging and the patent process for related therapies. During this course, students will learn how to (1) read and critically evaluate the primary research literature, (2) determine key mechanistic components of cellular immortality, (3) use experimental methods (including cell-lineage tracing, histology, protein biochemistry, and multiple Next Generation Sequencing technologies) to evaluate cell state and identity, (4) share their findings and opinions with peers, and (5) design experiments to test their own hypotheses.
Spring 2024
7.341 Your Brain on Bugs: The Gut-Microbiota-Brain Axis and Its Impact on Addiction
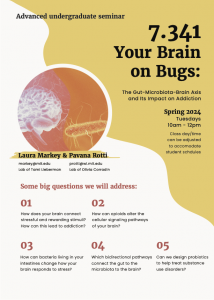
Instructor: Laura Markey (laboratory of Tami Lieberman) and Pavana Rotti (laboratory of Olivia Corradin)
From the moment of birth, humans are colonized by bacteria. By adulthood the gastrointestinal tract (gut) microbiota is made up of billions of bacterial cells, breaking down the food we eat and producing all sorts of compounds that interact with human cells and change their functions. Recent studies have shown that the gut microbiota influences how neural circuits in the brain change in response to environmental stresses. In this discussion-based course we will analyze primary research papers to learn how the gut microbiota signals to the brain and can influence human behavior through pathways that define the gut-microbiota-brain axis. Specifically, we will discuss how the gut-microbiota-brain axis can regulate stress responses ranging from sugar cravings to the development of substance abuse. The ability of the microbiota to impact this range of reward-related behaviors plays an important role in the development and severity of substance use disorders. We will start by learning about the basic brain regions and circuits involved in the normal response to stress and reward, and then how these circuits are impacted in the phases of addiction. Research into the molecular and cellular changes observed in neurons as a result of exposure to opioids and alcohol will be discussed. Then we will learn about the neuroendocrine, immune and metabolic pathways that connect the gut microbiota to the brain and how changes to the microbiota can change the stress and reward responses. We will read papers exploring how scientists are manipulating the gut microbiota, with traditional or engineered probiotic bacteria, to regulate the gut-microbiota-brain axis and treat substance use disorders. Students will learn about how animal and cell culture models are used to investigate complex disorders and the limitations of these models. Each session we will discuss two primary research papers. Students will use their critical thinking skills and learn how to dissect a research paper, interpret data, and place the findings of a single study into the context of the field. We will take a field trip to MarvelBiome, a biotechnology startup with ongoing clinical trials using probiotic therapy to treat neurodegenerative diseases. Students will be responsible for one main written assignment (a short original research proposal) and one final oral presentation, leading the class in a discussion and critique of a paper not previously considered in class.
7.342 Evade, Attack, and Multiply: How Pathogens Survive and Prosper in a Hostile World
Instructor: Dylan Valleau and Haley Licon (laboratory of Sebastian Lourido)
Envision a life as a pathogenic microbe. For successful infection, you must find a host, invade, replicate, avoid destruction by the immune response, and after all that, disseminate through a population to do it again! How will you pick which hosts to enter? How will you get there? And how do you ensure your own transmission before the host succumbs to your very presence? To answer these and other questions, we will dive into the riveting world of host-pathogen interactions. In this class, we use the primary literature to examine how microbes overcome, exploit, and manipulate host biology to promote their own survival. Drawing on studies from the fields of bacteriology, virology, and protozoology, we will explore the commonalities and differences in strategies used by diverse pathogens to establish infection, and the evolutionary basis of the systems involved. Students can expect to learn about the unusual biology of the parasite Trypanosoma brucei, which decorates its surface with a rotating array of decoy proteins to hide from the host immune system. Or the intracellular bacterium Listeria monocytogenes, which hijacks host actin to move through the host-cell cytoplasm and burst into neighboring cells. As we dig into the literature on these and other microbes, students will learn principles of experimental design, data analysis and interpretation, and effective data presentation. We will discuss classical and emerging techniques in biomedical research, including genetic engineering, next-generation sequencing, high-throughput screening, and quantitative proteomics. We will also visit the laboratory of MIT’s own Dr. Sebastian Lourido, where research centers on the protozoan parasite Toxoplasma gondii. Students will view these microbes by live fluorescence microscopy, observe demonstrations of several assays used to study Toxoplasma in the lab, and engage with scientists conducting this work first-hand. By the end of this class, students will have developed critical skills in reading and evaluating scientific literature, as well as an appreciation for the ingenuity, complexity, and resilience of the diverse microbes that impact human health.
7.343 How Cells Build Protein Networks that Drive Cellular Movement and Determine Cell Shape, and How these Networks Are Co-opted by Pathogens
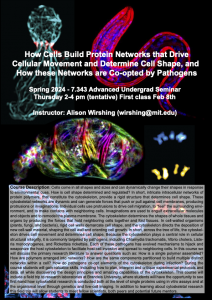
Instructor: Alison Wirshing (laboratory of Daniel Lew)
Cells come in all shapes and sizes and can dynamically change their shapes in response to environmental cues. How is cell shape determined and regulated? In short, intricate intracellular networks of protein polymers, that constitute the cytoskeleton, provide a rigid structure that determines cell shape. These cytoskeletal networks are dynamic and can generate forces that push or pull against cell membranes, producing protrusions or invaginations. Individual cells use protrusions to drive cell migration, to “feel” the surrounding environment, and to make contacts with neighboring cells. Invaginations are used to engulf extracellular molecules and objects and to remodel the plasma membrane. The cytoskeleton determines the shapes of whole tissues and organs by producing the forces that hold neighboring cells together and fold tissues. In cell-walled organisms (plants, fungi, and bacteria), rigid cell walls demarcate cell shape, and the cytoskeleton directs the deposition of new cell-wall material, shaping the cell wall and orienting cell growth. In short, across the tree of life, the cytoskeleton drives cell movement and determines cell shape. Because the cytoskeleton plays a central role in cellular structural integrity, it is commonly targeted by pathogens, including Chlamydia trachomatis, Vibrio cholera, Listeria monocytogenes, and Rickettsia rickettsia. Each of these pathogens has evolved mechanisms to hijack and weaponize the host cytoskeleton to facilitate host cell invasion and spread to neighboring cells. In this course we will discuss the primary research literature to answer questions such as: How is a single polymer assembled? How are polymers arranged into networks? How are the same components partitioned to build multiple distinct networks within a cell? How do pathogens co-opt elements of the cytoskeleton during infection? During the course students will gain valuable skills, including how to plan, interpret and critique experimental protocols and data, all while discovering the design principles and amazing capabilities of the cytoskeleton. This course will include a field trip to research laboratories at Brandeis University, where students will have the opportunity to see first-hand how cytoskeletal research is conducted both at the level of single proteins using in vitro assays and at the organismal level through genetics and live-cell imaging. In addition to learning about cytoskeletal research this field trip will allow students to meet fellow scientists, both peers and potential future mentors.