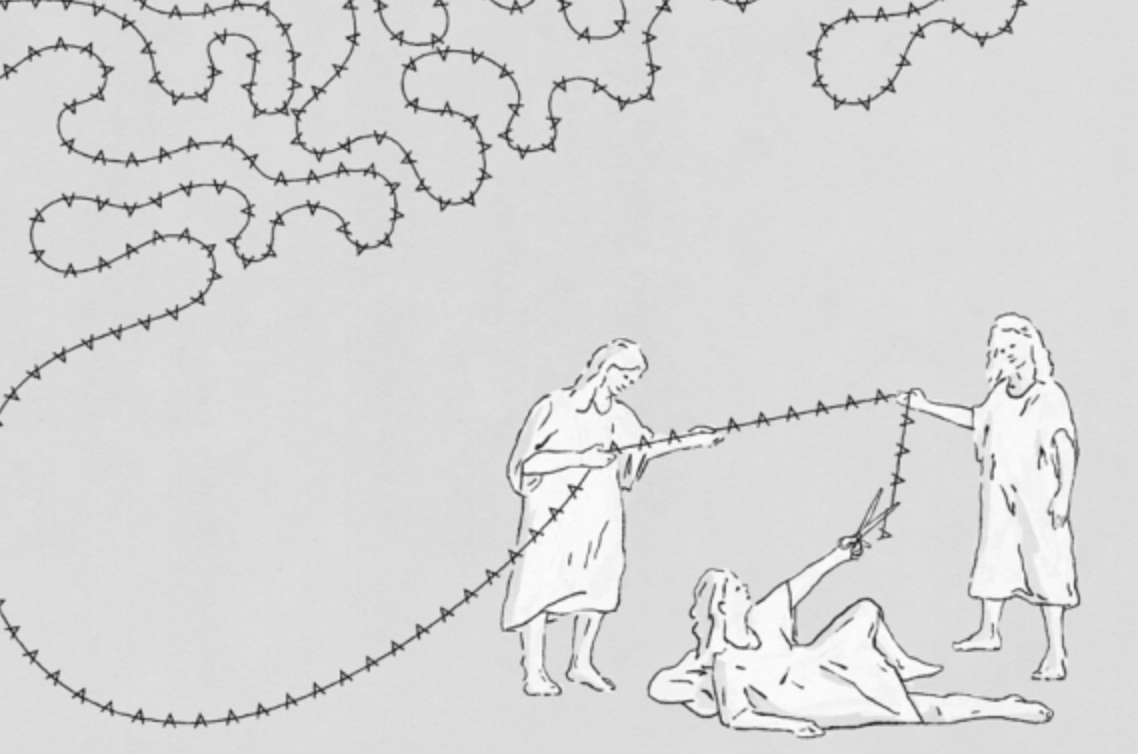
Nicole Davis | Whitehead
February 20, 2020
Genes are often imagined as binary actors: on or off. Yet such a simple view ignores the fact that genes’ activities, exerted by their corresponding proteins, can run the gamut from barely perceptible to off the charts. This rheostat-like range is due in part to molecular controls that determine how long the protein-making instructions for any given gene — known as messenger RNA (mRNA) — can persist before being destroyed.
Now, in a pair of papers published online in Molecular Cell, Whitehead Institute member David Bartel and his colleagues take a deep and systematic look at the dynamics of mRNA decay across thousands of genes. Their analysis — the most extensive to date — reveals surprising variability in the rate at which the ends (or “tails”) of mRNAs are shortened. In addition, the researchers uncover a link between this rate of shortening and how quickly the short-tailed mRNAs decay.
“Ultimately, these dynamics are responsible for determining how much mRNA is present for each gene, and that, of course, is really important for determining cell identity — for example, whether a cell is cancerous or a normal, healthy cell,” says Bartel, who is also a professor of biology at the Massachusetts Institute of Technology and an investigator at the Howard Hughes Medical Institute. “There is a thousand-fold difference in how long mRNAs stick around. That has a very profound effect on the amount of protein that gets made.”
TOWARDS A GLOBAL VIEW OF MRNA DEGRADATION
The anatomy of a typical mRNA consists of three key parts: a body, which contains the protein-making instructions; at one end, a string of repeating A’s known as the poly(A) tail; and at the other end, a protective biochemical cap.
Prior to the Molecular Cell studies, the future of a mRNA was known be linked to the length of its poly(A) tail — the longer the string of A’s, the longer the mRNA tends to persist. However, the speed that tails shorten as they age, and the rate at which mRNAs decay when their tails become short was known for just a handful of mRNAs.
To gain a more global picture, Bartel and his team, most recently led by graduate student Timothy Eisen, combined a set of techniques for high-throughput analyses of mRNA. These include a method for chemically modifying mRNAs as they are being made in order to distinguish newly synthesized mRNAs from those that are older, as well as sequencing-based approaches for measuring both the length of poly(A) tails and the amount of mRNA that was recently made. In addition, Eisen used computational methods to model the data they gathered and make predictions about them.
“All of the work in these papers involves time as an axis,” says Eisen. “The power of our approach is that it allowed us to plot and visualize how things change over time — and to infer for mRNAs from thousands of genes the rate at which the tail shortens and the subsequent rate at which the mRNA is destroyed.”
THE TAIL WAGS THE MRNA
By leveraging these techniques, Bartel, Eisen and their colleagues explored the mRNA dynamics for thousands of genes. One key observation is that mRNAs enter the cytoplasm with diverse poly(A) tail lengths. That variability encompasses not only the mRNAs from different genes but even those that correspond to the same gene.
“Previously, there wasn’t any reason to think there would be any differences, so people just assumed that the initial tail lengths would be the same,” says Bartel. “But it turns out there’s quite a bit of variability there.”
The Whitehead team also uncovered a striking amount of variation in the rate at which poly(A) tails are shortened. For some mRNAs, the tail shortens at a rate of about 30 nucleotides per minute. With an average tail length of around 200 nucleotides, that translates to the tail lasting just a few minutes. Other mRNAs have much more durable tails, with shortening rates of just a nucleotide or two an hour.
“That’s a thousand-fold difference,” says Eisen. Previously, researchers had shown that tail-shortening rates could vary, but they had observed only a 60-fold difference.
Bartel and his colleagues also found some striking differences among mRNAs once their poly(A) tails became short. “If we consider just those mRNA molecules that have tails of only 20 nucleotides, the ones that come from certain genes disappear much more rapidly than those coming from other genes — again spanning a thousand-fold range,” says Bartel.
That finding challenges long-held views about mRNA stability, as it had been generally assumed that short tails equaled short lives, and that all mRNAs whose tails had been shortened decay at the same rate. But it turns out that both processes are important: the rate at which mRNA tails are shortened (a process known as deadenylation), and the rate at which mRNAs decay after this shortening. Moreover, Bartel and his colleagues find that these two processes are coupled — the more rapidly deadenylated mRNAs also degrade more rapidly once they have short tails.
“This coupling between rate of decay of short-tailed mRNAs and the rate of deadenylation is important because it prevents a large build-up of short-tailed versions of mRNAs that had undergone rapid deadenylation,” says Bartel. “Because these short-tailed versions do not build up, the thousand-fold difference that we observe in deadenylation rates can impart a thousand-fold difference in mRNA stabilities.”
SHINING A LIGHT ON MICRORNAS
MicroRNAs are small, regulatory RNA molecules that play critical roles in human biology. Their primary job is to recruit molecular machinery that shortens the poly(A) tails of mRNAs, thereby accelerating mRNA degradation, which reduces gene activity.
But strikingly, when Eisen and his colleagues harnessed their elegant system to examine microRNA activity, it appeared that these regulatory RNAs were leaving the tails of their targets completely unaltered — despite the fact that those mRNAs were being more rapidly degraded.
“That really left us scratching our heads wondering, ‘How could this be?’” adds Eisen. “It’s been known for quite some time that microRNAs operate by influencing poly(A) tail length.”
The team decided to look at the dynamics of this process, focusing on newly generated mRNAs. In this context, they observed that microRNAs accelerate both tail-shortening of target mRNAs and the subsequent decay of those mRNAs once their tails become short. “This second aspect of microRNA activity really hadn’t been appreciated before,” says Bartel. “But it’s a critical part of the story because it helps explain why we don’t see a build-up of short-tailed mRNAs.”
These findings, as well as the other results described here, significantly enhance what is known about mRNA decay and the factors that can influence it. With this expanded knowledge, Bartel and his colleagues, together with other research teams can work to uncover the molecular components and cellular contexts that cause mRNAs to have such drastically different lifetimes.
***
Written by Nicole Davis
***
Citations:
Eisen T, et al. The Dynamics of Cytoplasmic mRNA Metabolism. Molecular Cell. Published online January 2, 2020.
Eisen T, et al. MicroRNAs Cause Accelerated Decay of Short-Tailed Target mRNAs. Molecular Cell. Published online January 2, 2020.