Stretch and relax: Cells coordinate divisions during development to fold tissues
Lucy Jakub
Consider the fruit fly, Drosophila melanogaster. Though it’s only a couple of millimeters long, its body is intricately complex. But it began, as most animals do, as an amorphous blastula—a hollow ball of dividing cells. During embryonic development, the structures of the body emerge as cells multiply and change shape, sculpting tissues into the mature forms dictated by the genetic code. One of the first structural changes is gastrulation, during which the blastula becomes multilayered with an ectoderm, mesoderm, and endoderm. In the developing fly, this occurs through a tissue folding mechanism. The first fold is the invagination of the mesoderm, when cells fated to become muscles contract and curl inward, leaving the cells fated to become skin on the exterior.
Biologists have traditionally focused on how cells generate force to understand cell and tissue shape change. But researchers at MIT have found that there’s another important, though often overlooked, player in tissue folding: cell division, or mitosis. By combining live-imaging with genetic mutations of developing Drosophila embryos, they observed that cell constriction and division can act together to promote folding, and that mitosis interferes with the accumulation of motor proteins that allows cells to generate force.
“What the results tell us is that the cell cycle and cell division might need to be tightly regulated relative to other shape changes that are happening in the tissue,” says Adam Martin, the senior author of the study published on March 13 in Molecular Biology of the Cell. “They present a new paradigm for thinking about how tissue shape might be regulated during development, and provide insight into what might cause birth defects in humans.” Clint Ko PhD ’20, a former graduate student in the Martin lab, was lead author of the study.
In 2000, three different labs identified a genetic mutation that caused premature cell division in developing Drosophila embryos. They found that the gene tribbles, named for the fuzzy, rapidly-reproducing animals in Star Trek, regulates cell division in the mesoderm of the fly, ensuring that cells only divide at the appropriate time. When that gene is deleted, cell division occurs before the mesoderm can properly internalize. What was notable about this mutant was that the blastula never folded, and remained a ball of cells instead of an envelope of tissue with an inside and an outside. This observation led researchers to believe that cell cycle regulation somehow regulates tissue folding. But, at the time, there was no live-imaging technology to visualize how cells changed in the developing embryo.
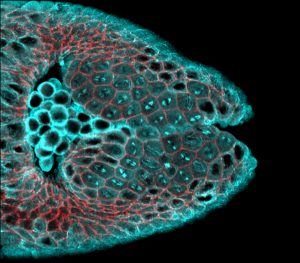
Specifically, researchers in the Martin lab wanted to see what was happening to networks of the motor protein myosin, which allows cells to contract, in the tribbles mutant. Myosin is the same protein that allows our muscle tissue to contract when we flex. To facilitate tissue folding in the developing fly, myosin is concentrated at the top of the cells in the mesoderm, where they form the surface of the blastula. As this myosin constricts, the outer surface of the tissue shrinks and contracts inward.
By using a fluorescent protein to visualize chromosome condensation, which marks the start of mitosis and the cell’s preparation for division, the researchers were able to use live-cell imaging to see how premature division might be interfering with cell constriction. When a cell prepares to divide, it expands and becomes rounded, before elongating—shape changes that exert force on neighboring cells. But something else was going on, too.
“We noticed that when the cells are dividing, the apical myosin networks that are present disappear,” says Ko. Cells that had already begun to contract relaxed when they entered mitosis, indicating that it’s a loss of contractility in the tribbles mutant that prevents folding. The researchers suspect that this reversal occurs because mitosis disrupts signaling from the gene RhoA, which regulates contractility and cell shape changes during development. An undergraduate researcher in the lab, Prateek Kalakuntla, showed that regulation of RhoA changes at the start of mitosis.
“Initially we were just curious about the tribbles mutant,” says Ko. “But then we started exploring other ways of looking at how cell divisions affect myosin accumulation in cells.” They utilized a mutation in which the gene fog, which is located upstream of myosin activation on the genome, was overexpressed. (Fog is short for “folded gastrulation.”) Cells in the Drosophila ectoderm don’t normally contract, but with ectopic fog overexpression, those cells activated myosin, too. With live-cell imaging, the researchers observed furrows develop across the ectoderm.
“It was a bit unexpected to see these tissues folding when they shouldn’t be folding,” says Ko. Specifically, the folds occurred along the boundaries of mitotic domains, regions of spatiotemporally patterned cell divisions that occur in coordinated pulses. “That led to this sort of novel idea that cell divisions—particularly when they’re in this pattern where they’re interspersed between contractile cells—can actually promote tissue folding.”
Understanding the genetic basis for tissue folding, and how our genes control the development of specific bodily features, can help determine how birth defects arise during development. “If cell cycle control is misregulated during development, it could actually alter the shape of that tissue,” says Martin. The study paves the way for further research into how exactly the location of myosin in the cell is regulated, and how it is affected at the molecular level by cell division.
“We observed that when these cells enter mitosis, the localization of myosin activators changes. But we don’t really know how it changes,” says Ko. “That would be a pretty interesting research problem, especially considering that it’s such an integral part of force generation in cells.” Kalakuntla has begun investigating what controls these regulators, which will be an avenue of future research for the lab.
Top image: Myosin networks, in green, contract cell membranes in the mesoderm of a developing Drosophila embryo. Credit: Martin lab.
Citation:
“Apical Constriction Reversal upon Mitotic Entry Underlies Different Morphogenetic Outcomes of Cell Division”
Molecular Biology of the Cell, online March 4, 2020, DOI: 10.1091/mbc.E19-12-0673
Clint S. Ko, Prateek Kalakuntla, and Adam C. Martin