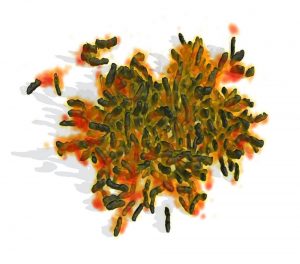
Jörn Dunkel’s group in the MIT Department of Mathematics teams up with bench scientists to understand how bacteria interact with each other and their environments.
Saima Sidik
Saima Sidik is a research associate in the Lourido lab at the Whitehead Institute. This piece is part of a departmental effort to highlight biologically relevant research being done outside the traditional life science disciplines and departments at MIT.
“The crux of the issue.” “The root of the problem.” “The truth of the matter.” So often we want to distill substances, systems, or life, down to a single essence: a chemist isolates key compounds from complex mixtures; a writer looks for the kernel of truth hidden in a story; and a yogi meditates to find inner meaning. In the MIT Department of Mathematics, Jörn Dunkel turns to math to uncover the fundamental components of physical processes. His group has recently identified key features that regulate bacterial growth, findings that could eventually reduce the frequency of hospital-acquired infections, make antibiotics more effective, and even create self-healing materials.
Dunkel’s group creates mathematical models of physical systems as they change through time and space. They’ve studied elastics buckling, pasta breaking, and, most recently, bacterial communities growing. One might not expect to find a group of mathematicians immersed in biology, and yet the Dunkel group has made great strides towards understanding the process in which bacteria give up their ability to swim, attach to a solid surface (like the hull of a boat or the lining of a digestive tract), and divide until they build a mound called a “biofilm.” Biofilms protect the cells within them, allowing those cells to survive immune pressure, antibiotics, and environmental stresses, which makes biofilm formation critical for some bacteria to survive under harsh conditions. By understanding the factors that drive these pathogenic structures, researchers can find ways to limit biofilm formation.
In collaboration with Knut Drescher’s lab at the Max Planck Institute for Terrestrial Microbiology, the Dunkel group builds mathematical models that describe how biofilms develop. The Drescher lab takes high resolution videos of biofilms growing over the course of multiple hours, and then they pass these videos off to the Dunkel lab. The mathematicians sift through the videos, extracting every kind of information that might be biologically relevant: the cells’ orientations, movement, rate of division, the distance between cells, and many other features.
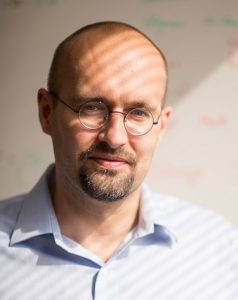
It’s only recently that advances in biofilm imaging have allowed researchers to see individual bacteria within biofilms and measure the features that the Dunkel group uses in their models. “You get such rich data sets that traditional methods of building models become infeasible,” Dunkel says. “Ten years ago, it wouldn’t have made sense to think about these questions because we didn’t have the technology to answer them.”
With such extensive data, the challenge that the mathematicians now face is figuring out how to describe the behavior of biofilms in terms of a minimal set of their many features. And so, with a cut-throat efficiency that would make the organizing guru Marie Kondo proud, they line up all the measurements they can make, then prune away any that aren’t clarifying the system, until they’ve identified the minimal number of features that can explain the behavior of the bacteria. Their results indicate that the attractive forces between bacteria, along with the spatial constraints that they experience when they reproduce, are essential in shaping biofilm development.
The Dunkel group hopes their work will help limit hospital-acquired bacterial infections. Medical devices like pacemakers or catheters can become homes for these bacterial accumulations if these devices come into contact with dirty gloves or water. From there, infections can spread and cause blood, urinary, skin, and lung infections, among other problems. Because the bacteria at the centers of biofilms are shielded from antibiotics, these infections can be very difficult to treat. The Dunkel lab’s models reveal that simply changing the shape of a medical device may make it less likely to support biofilm formation, or make the biofilms it harbors more susceptible to antibiotic treatment.
Phillip Pearce, an applied mathematics instructor who works with Dunkel at MIT, is leading a branch of the group’s research that’s focused on how biofilms develop when liquid moves over them: a situation that’s central to understanding biofilms in medical devices where liquids like blood and urine frequently flow past. He explained how he and the rest of the Dunkel group started with a list of biofilm features and derived models that identified the physical processes that are necessary to explain these observed features.
When bacteria divide, the new bacteria need someplace to go. This presents a problem for bacteria when they’re anchored in place, as they are in the middles of biofilms. Since these bacteria can’t always spread horizontally, spatial constraints force them to stand on end, making the biofilm grow vertically. This results in a very organized structure with the internal bacteria sticking upwards, like slats in a picket fence. After examining many combinations of biofilm features in their models, the Dunkel group realized that the forces bacteria put on each other when they grow play a major role in dictating this picket fence arrangement. Bacteria at the front of the biofilm are also forced into a vertical orientation, enhancing the regular organization of the biofilm.
By redesigning medical devices to include complex shapes, researchers might be able to limit the ability of any bacteria that colonize them to display such regular arrangements. This might make biofilms more susceptible to antibiotic treatment, or it may prevent them from forming in the first place.
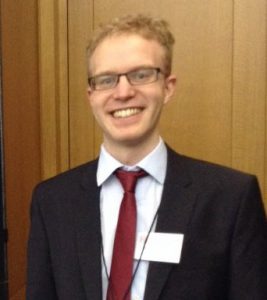
While some members of the Dunkel group are trying to thwart biofilm formation, Boya Song, a graduate student, is investigating situations in which biofilms can be useful. Part of what holds these structures together is a sticky matrix of proteins, sugars, and fats that the bacteria secrete. The Dunkel group thinks that if they can manipulate the shape of biofilms, they can also manipulate the shape of this matrix.
“Biofilms could be used to form new biomaterials based on the lattice shapes that the cells produce,” Song says. These materials could form protective linings for the hulls of boats or for submerged metals. Some bacteria deposit minerals, and these could be programmed to repair bone or even fix cracked cement.
Dunkel is excited about this possibility. “Maybe if we understand biofilm formation, we can actually program them!” he says.
Understanding how to program biofilms involves understanding surface proteins called “adhesins” that act like hooks to hold bacteria together. The Dunkel group’s models show that when bacteria are in nearly stationary liquid, the adhesive forces generated by these surface proteins largely dictate the size of a biofilm and the arrangement of the cells within it.
Rachel Mok, another graduate student in the Dunkel group, took their adhesion model for a test drive by applying it to bacteria that express varying levels of an adhesin in response to varying concentrations of a drug. Mok tuned her model to account for this experimental manipulation of the attractive force between bacteria, and she found that she could predict how the bacteria would behave.
“It’s pretty incredible that even though we’ve neglected a lot of biological features, like nutrient availability, that we can still capture the dynamics that we see in the early-stage biofilms,” she says.
In addition to altering adhesin levels, the Dunkel group has collaborators in the Riedel-Kruse lab at Stanford University who can vary how the adhesins work. Typical adhesive proteins can either grab other proteins, or they can be grabbed. By bestowing genetically altered adhesins upon bacteria, they’ve been able to split these two functions, resulting in one group of bacteria that can only grab others, and one that can only be grabbed. By mixing these two groups in different proportions, they can manipulate the shape of the resulting biofilms.
Song plans to combine the Dunkel group’s knowledge regarding adhesins with their collaborators’ findings to gain even more control over biofilm shape. Perhaps one day the technology they create together may allow them to use bacteria to “print” materials in any shape they choose. Because the bacteria within a biofilm can secrete additional material if the matrix is damaged, this would result in a programmable, living, and self-healing building material. The models Song is developing are an early step in this ambitious project, which has the potential to change the face of materials science.
From high-resolution microscopy to bacterial genetics to mathematical modeling, figuring out biofilms is an inherently interdisciplinary problem. The Dunkel group has identified essential features that define biofilm development, and their models predict how these structures develop, whether on the hull of a boat or in the lab. By putting their minds together, they and their collaborators are re-writing rules for approaching biofilms, be it to prevent their growth or to harness it.
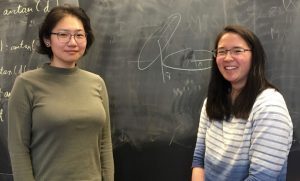