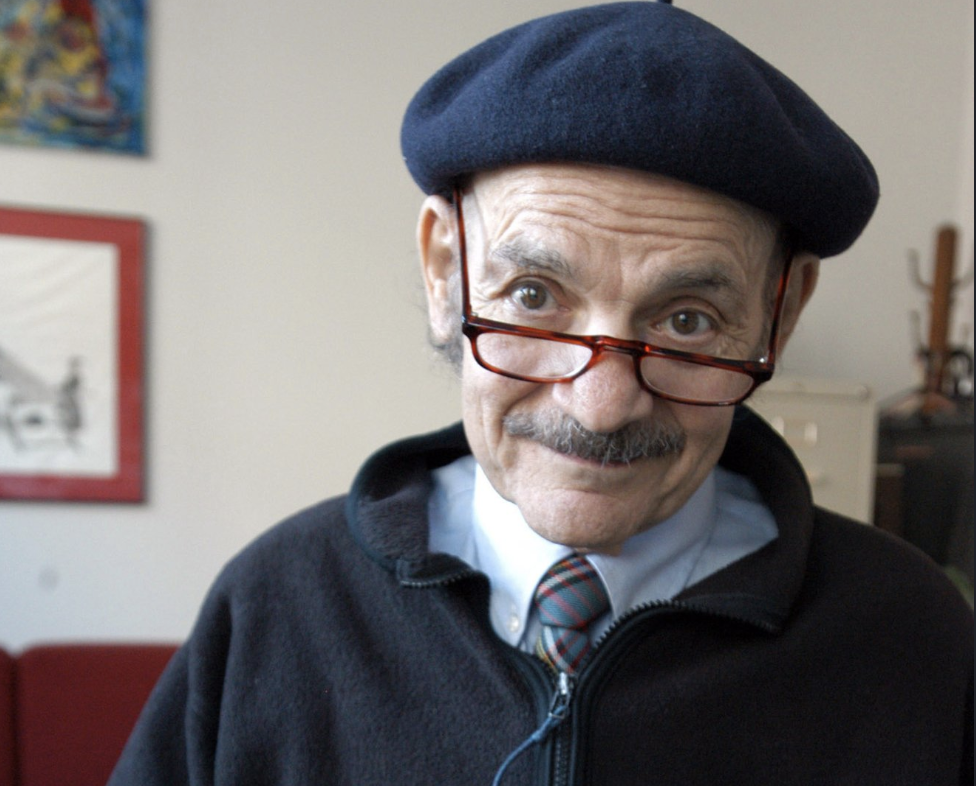
Chris Kaiser | School of Science
May 31, 2018
Alex Rich had a long and fertile career at MIT working on the relationship between the molecular structure and the function of biological information molecules DNA and RNA. Rich is perhaps best known for the elucidation of the three-dimensional structure of a transfer RNA molecule, and for the discovery of an alternative form of DNA that exists in certain biological contexts, known as Z-DNA.
Less well-recognized is Rich’s contribution to the discovery of nucleic acid hybridization. Hybridization is the process by which single-stranded RNA or DNA molecules can find each other in solution by the exact matching of complementary base sequences. The rate of hybridization is limited only by the rate of diffusion of molecules in solution. Because of its remarkable speed and specificity, hybridization remains today as one of two fundamental methods for reading out the identity of RNA or DNA molecules in different contexts — the direct determination of the base sequence, with the other being carrying out the matching by computer.
Rich grew up in a working-class neighborhood of Springfield, Massachusetts. In high school, he helped support his family by working in the U.S. Armory machining grooves in rifle barrels. As a young man, Rich was smart, resourceful, and ambitious and he received a fellowship to Harvard College and later attended Harvard Medical School. At Harvard, Rich had the opportunity to work with Professor of Biological Chemistry John Edsall, who sparked an interest the physical chemistry of biological macromolecules that eventually led him away from medicine to postgraduate research with the visionary chemist and ebullient polymath Linus Pauling at Caltech.
Pauling discovered the alpha helix as a basic element of protein structure and by doing this invented the method of model building as a way of predicting the large-scale structural features of complex macromolecules from the chemical bonding structures of their constituent parts. In Pauling, Rich found a powerful role model who showed by example how far you could travel by grasping a good idea or deep insight.
When Rich joined the lab, Pauling was working on a structure for DNA and then was, to put it bluntly, scooped by James Watson and Francis Crick. Watson and Crick’s structure for DNA, based on astute model building and the X-ray diffraction data of Rosalind Franklin, was published in 1953. The key feature of their structure was the exact pairing of the bases between two strands of DNA that twist around each other in a double helix. The base-pairing rules — adenine pairs with thymine and guanine pairs with cytosine — are imposed by the geometric constraints on the paired bases as they are held together by hydrogen bonds in the central core of the helix. The double helix can accommodate a string of bases of any sequence and thus carry genetic information encoded in linear sequences of four characters. Moreover, the exact base-pairing between strands means that each strand carries the same information as the other, but in complementary form, and immediately suggested how the genetic information can be duplicated for cell division.
Big Bang and the coding problem
The emerging picture that the base sequence of DNA carried instructions to synthesize linear strings of protein out of a set of 20 amino acids led to a deeper puzzle: how could information encoded in the sequence of one kind of macromolecule be translated into the sequence of an entirely different kind of molecule? Although its direct involvement had not yet been shown, RNA was strongly suspected to have a central role in this process. One of the clues to the involvement of RNA was that RNA was most abundant in animal or plant tissues undergoing rapid growth and therefore extensive new protein synthesis. DNA and RNA are similar molecules and both are polymers of four nucleotide bases, but they differ in that DNA contains a hydrogen atom at the 2’ position on the ribose ring, whereas RNA contains a hydroxyl group at this position. The absence of a hydroxyl group at this position makes DNA more chemically stable and therefore more suitable to carry the permanent copy of genetic information. Also, DNA carries the base thymine instead of the chemically similar base uracil in RNA.
The brilliant theoretical cosmological physicist George Gamow, who was an early proponent of the Big Bang theory, saw that there was something worthy of interest in RNA and what soon became known as the “coding problem.” Gamow helped to focus thinking about this problem by posing the question of how a code written in four bases could be translated into 20 different amino acids. The introduction of the principles of information theory, first proposed in Claude Shannon’s 1948 paper “A Mathematical Theory of Communication,” immediately suggested that at least three bases would be required to carry enough information to specify 20 different amino acids. Gamow organized interested scientists in a group that called themselves the RNA Tie Club — so named for members’ necktie clips that bore the abbreviation of an amino acid. Rich was a member, as were Watson and Crick; and these members would share with one another ideas and insights before publication. Since each member of the club was assigned a different amino acid, membership never exceeded 20.
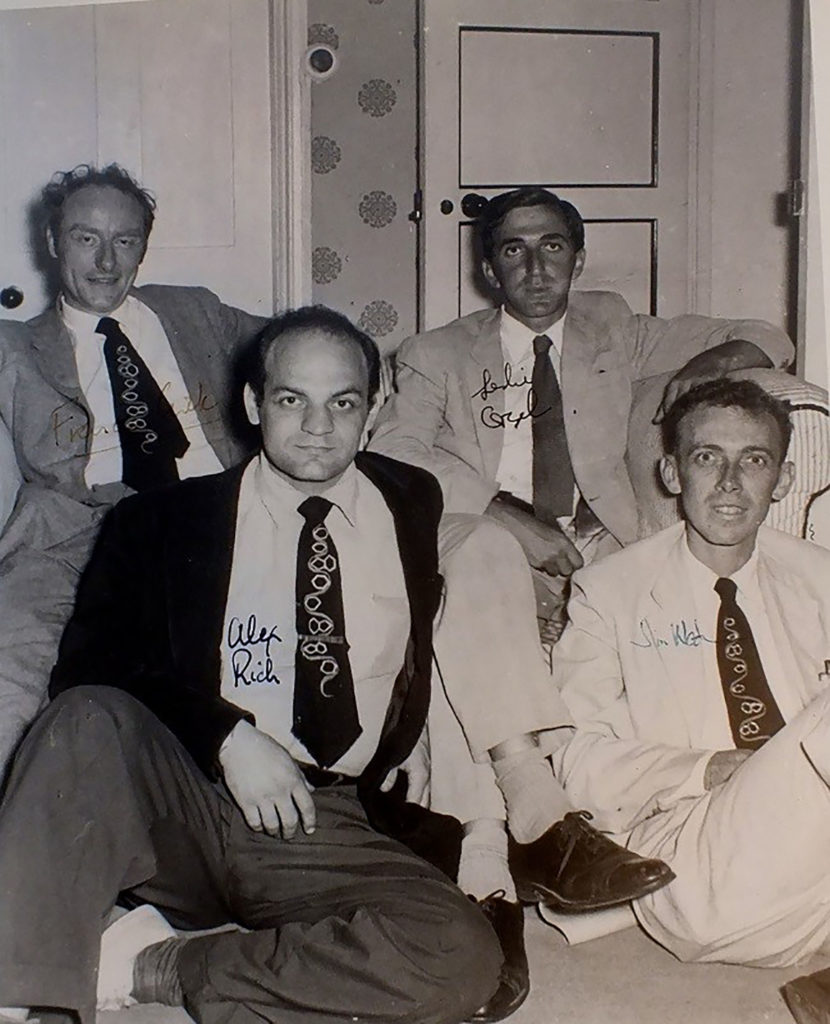
Rich was captivated by the connection, as so beautifully illustrated by the DNA model, between chemical structure and biological function and he was determined to make his mark in this new field as a structural biologist. He wondered if RNA could form a double helical base-paired structure and what role might this structure have in translating a DNA code into amino acids. With the help of Watson, who was at Caltech at that time, Rich set about analyzing different kinds of natural RNA samples, but none showed the characteristic diffraction pattern in X-ray analysis that Franklin had seen for double helical DNA.
Rich took a job at the NIH. There and on a sabbatical to Cambridge, England, he had success with various structural and modelling studies, including a structure for collagen, but he kept coming back to the question of whether a double-stranded RNA helix could form. One of the most precise analytical tools for nucleic acids such as RNA available at the time was to measure the base composition — that is, the relative proportion of guanine, cytosine, adenine and uracil. For a fully double-stranded molecule, base pairing rules would dictate that the amount of guanine should equal cytosine and the amount of adenine should equal that of uracil. The natural RNA samples that Rich was studying had very different base compositions, but did not follow the rules expected for a double-stranded structure. Eventually, Rich decided to force the issue by synthesizing his own RNA molecule that could form a fully base–paired double strand.
Rich and his colleague David Davies used the enzyme polynucleotide phosphorylase, which could polymerize into chains whatever activated nucleotide precursors were provided, to prepare two RNA chains designed to be able to base pair with each other. In one reaction, they prepared a long strand of RNA with only adinines (oligo-A) and in a separate reaction a long strand of RNA made up entirely of uracil (oligo-U). Hoping to see some amount of base pairing, Rich mixed the two preparations together and was amazed to see the entire contents become converted into RNA with the properties of double-stranded molecules. X-ray analysis confirmed that the two chains had had coiled around each other into a double helix. This experiment showed that an RNA-based double helix was possible, but the speed with which the double-stranded molecules formed was entirely unexpected. Based on physical chemistry of polymers, Rich had expected that some additional factors, such as enzymes, would be needed to neatly coil long disordered chains around each other. The effect of seeing this dramatic reorganization of molecules happen so efficiently might be the equivalent of seeing two tangled fishing lines that were thrown together spontaneously wrap themselves into a neat braid.
Such spontaneous base pairing between different nucleic acid chains is known as hybridization and is the fundamental underlying chemical process by which the information in DNA is translated into protein. Rich went on to show that hybridization between a DNA strand (oligo-dT) and an RNA strand (oligo-A) could occur to form a hybrid of RNA based paired with DNA. This molecule provided a structural basis for copying information from the gene sequence in DNA into a complementary single-stranded messenger RNA molecule. Moreover, base pairing between triplet codons on the messenger RNA and the anticodon loop of a transfer RNA carrying a specific amino acid is the basis by which the nucleotide code is translated into amino acid sequence.
Hybridization has become an enduring method in molecular biology and biotechnology research. Shortly after Rich carried out his RNA hybridization reaction, it was shown that the two strands of DNA could be melted apart at high temperature and then could come back together in a sequence specific manner if held at a somewhat lower annealing temperature. Before methods for direct sequencing of DNA became available, hybridization was the only method by which specific DNA or RNA sequences could be identified in a complex mixture.
Hybridization has become an enduring method in molecular biology and biotechnology research. Shortly after Rich carried out his RNA hybridization reaction, it was shown that the two strands of DNA could be melted apart at high temperature and then could come back together in a sequence-specific manner if held at a somewhat lower annealing temperature. Before methods for the direct sequencing of DNA became available, hybridization was the only method by which specific DNA or RNA sequences could be identified in a complex mixture.
Hybridization was crucial for the discovery of splicing of messenger RNA made by MIT Institute Professor Phil Sharp and was the basis for Professor Susumu Tonegawa’s demonstration of DNA rearrangements that underlie the formation of functional genes for antibodies. Even now, with extremely powerful methods for DNA sequencing, hybridization is still often used to examine the structure of chromosomes and to conduct comprehensive studies of gene expression based on microarrays. Finally, sequence-specific hybridization is at the heart of natural processes that have been harnessed for RNA interference of gene expression and CRISPR-based genome editing.
Rich himself wrote and spoke extensively about the early years of molecular biology in ways that reveal two important characteristics as a scientist. The first is that his deep admiration for mentors such as Pauling and colleagues such as Crick and Watson was the basis of an intellectual network that sustained Rich his entire career. By his account, a new discovery in the lab was invariably followed by a letter or a phone call to those that he admired to get their reactions. All biologists, no matter how great, struggle with the problem that it is difficult — if not impossible — when setting out on a new problem to predict whether it will reveal insights fundamental to all living things or merely lead to odd details produced as a byproduct of the tinkering of evolution. Rich was adept at vetting new ideas through his constellation of brilliant friends to guide him toward the fundamental.
The second, related characteristic is Rich’s gift for seeing how new concepts may play out in time — well into the future. In the spirit of the RNA Tie Club, Rich freely shared his imaginative speculation about where he saw the field going, adding these forward-thinking ideas to his review articles and sprinkling their seeds in the discussion sections of his research papers. Among his more prescient ideas was the prediction that hybridization to messenger RNA of a complementary regulatory RNA could play a part in gene regulation; this prediction anticipated the discovery of microRNA-based regulation by about 40 years. He also hypothesized in the early 1960s that early life forms could have a genetic system without DNA that was made up of only RNA. This may be the first articulation of the now widely accepted idea of an RNA world. As I knew Rich in his later years, he remained engaged in and stimulated by new ideas. It was not difficult when chatting with him in his office or going on a walk-and-talk with him to feel connected to and stimulated by the sweep of brilliant ideas that have propelled molecular biology along from the very beginning.