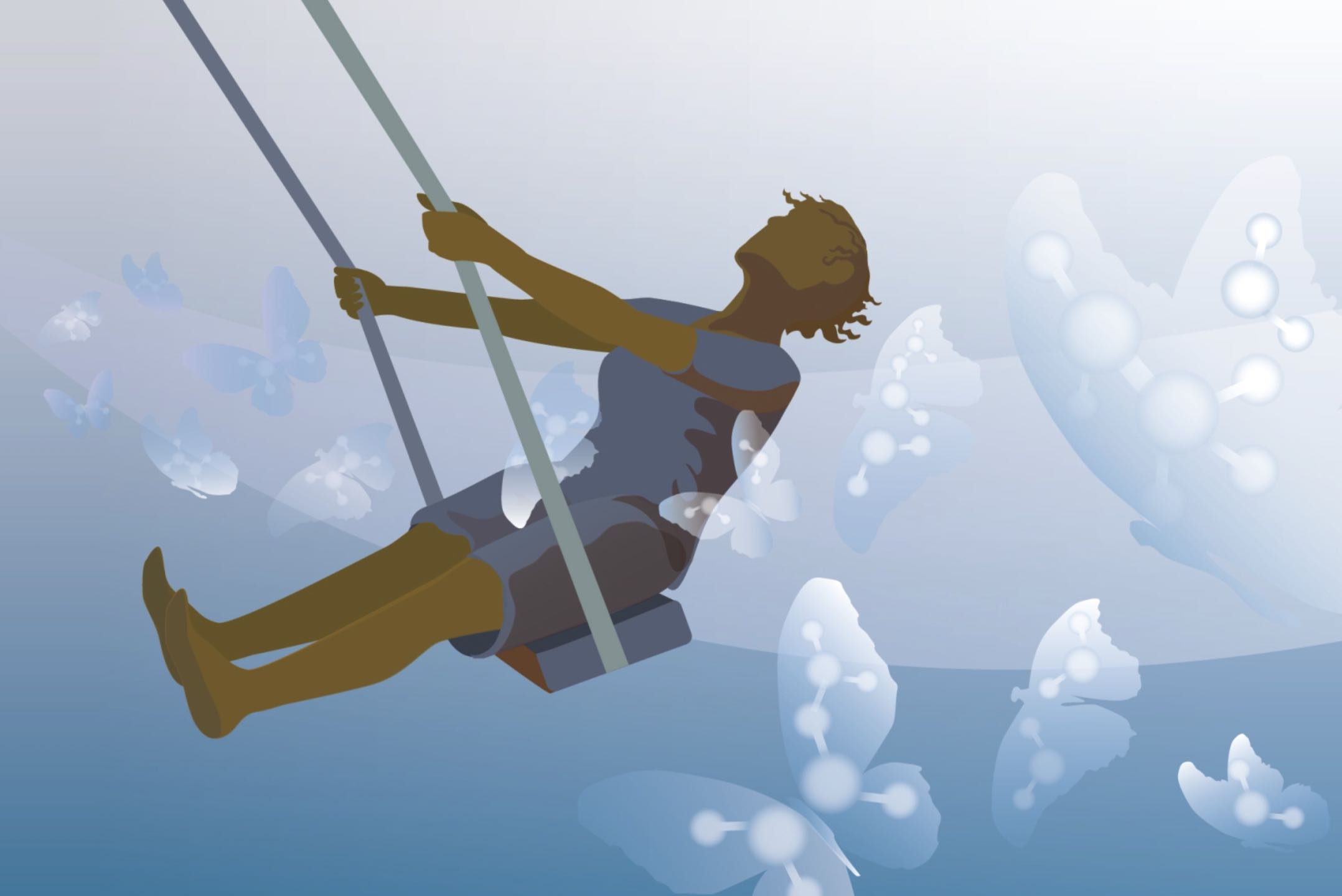
Whitehead Institute
March 25, 2022
For the hundreds of thousands of people diagnosed with breast cancer each year, surgery to remove the cancerous tissue is often the best option — but this relatively simple procedure comes with some drawbacks. In more than a few cases, the surgical removal of a tumor can lead to an increased risk of the cancer reemerging in other locations in the body.
In a 2018 study, a postdoc in the lab of Whitehead Institute Member Bob Weinberg discovered that, at least in mice, this phenomenon was due to a bodily butterfly effect: the creation of a wound site in one place in the body, which necessitated subsequent wound healing, caused immune system changes affecting distant parts of the body.
These changes occurred as bone marrow cells responded to the wounding with a flood of inflammatory cells that entered into the wound site and, at the same time, scattered throughout the body. These dispersed inflammatory cells weakened the ability of the immune system to control the outgrowth of a distantly located metastatic tumor. Without this immune control, which otherwise could keep the metastasis at a very small size, the metastasis would grow out aggressively.
Hence, wounding in one part of the body provoked metastasis outgrowth at a distant site. This suggested, among other things, that the outgrowth of metastatic tumors, which is often seen in women who have recently undergone a mastectomy, might be actively provoked by the post-surgical wound-healing process.
Weinberg’s work also presented a way to potentially avoid this effect, using a preventative measure that’s probably sitting in your bathroom cabinet right now: the cheap and common class of drugs known as NSAIDS, which includes ibuprofen and aspirin. When mice were given NSAIDS before and after tumor removal surgeries, they experienced a fivefold lower rate of cancer recurrence at the site of metastasis than a control group given opioids. These NSAIDs could therefore be used in place of the opioids, which are often used to treat post-surgical pain.
The human body is full of undiscovered connections like this one and adding in foreign substances further complicates matters. While a treatment might work well in a Petri dish, researchers describe whole -body metabolism as “a whole different kettle of fish.”
The way drugs move through the body and interact with internal systems is called pharmacokinetics. When a person is given a medicine — either orally, through a chemotherapy method, or via injection — that drug must be able to find its way to its target in a high enough concentration to have an effect, and then when its purpose is served, it must be able to leave the body safely and not build up to a harmful amount.
Much like Weinberg’s work on NSAIDS in breast cancer, Whitehead Institute’s basic research has led to other surprising discoveries about drug activities in the human body. Read on to learn about research that is changing the way new drugs are designed, making existing treatments less toxic, and more.
Concentration is key
When it comes to the action of drugs in the human body, concentration is key. Just ask Rick Young, a Whitehead Institute Member and professor of biology at MIT. In 2018, Young’s lab, which had previously studied the regulatory circuitry involved in transcription (the copying of DNA into RNA), shifted its focus after discovering tiny droplets within cells that concentrate the molecular materials needed to transcribe the DNA.
The droplets, called transcriptional condensates, were the newest in a slew of recent discoveries of other such groupings of cellular components. Some of these aggregations facilitate RNA splicing while others help to form ribosomes.
For Young, the discovery of transcription-related condensates sparked an interest in how these droplets were affecting the action of drugs. Previous theories held that transcription was able to take place in cells because there was a sufficient concentration of necessary proteins, such as RNA polymerase and other accessory proteins. As the Young lab showed, these collaborating cellular players were actually being concentrated in the condensates,
In 2020, Young and Ann Boija and Isaac Klein, two postdocs in his lab, took their investigation a step further, analyzing the mechanism by which several cancer drugs are concentrated in cellular condensates, and how that concentration could affect their action in individual cells and thus in the body. They found that cancer drugs sort themselves into specific types of condensates, independently of their targets, which can allow them to build up into high concentrations in these localized areas within cells.
“This could have enormous implications for the way we discover and develop drugs,” said Rick Young. “If drugs had properties that had them partitioning into a condensate where their target lives, then they would enjoy two properties of condensates: they would be compartmentalized, and they would be at much higher concentrations than if they diffuse through the cell.”
Young’s work on condensates led him to co-create a pharmaceutical company called Dewpoint Therapeutics, with the goal of reformulating treatments for cancer or neurological conditions such as amyotrophic lateral sclerosis by targeting biomolecular condensates. Whitehead Institute Founding Member Rudolf Jaenisch serves as a scientific advisor.
Trouble in parasites
While researchers in Young’s lab investigate how drugs could be more efficiently targeted, Sebastian Lourido’s lab is taking a different tack — why do some drugs stop working as time progresses?
The malaria drug artemisinin was developed in China in the 1970s, and completely changed the way the world treated malaria. In the following decades, however, the parasites that cause malaria, several species within the genus Plasmodium, have slowly grown less susceptible to the drug.
In a paper published in September of 2020, Whitehead Institute Member Lourido and collaborators identified two parasite genes that were negatively impacting the actions of the drug in the parasite’s cells.
Researchers liken artemisinin to a “ticking time bomb,” which needs another molecule, called heme, to light its fuse. Heme, a small molecule that is one component of hemoglobin, helps transport electrons and deliver oxygen to tissues. When heme encounters artemisinin, it activates the drug, allowing the creation of small, toxic chemical radicals. These proceed to react with the parasites proteins, fats, and metabolites, eventually leading to its death.
In order to understand how some parasites were becoming less vulnerable to the drug, Lourido, along with researchers Clare Harding, Boryana Petrova and Saima Sidik, ran a genetic screen on a related parasite, Toxoplasma gondii. The screen allowed them to assess which mutations in the parasites’ genomes were beneficial for their survival and which ones were harmful.
The screen revealed two genes that affected how susceptible the parasites were to treatment with artemisinin. One, called Tmem14c, seemed to be protecting the parasites. The gene is analogous to a gene that transports heme out of mitochondria where it is generated. Lourido hypothesized that when the Tmem14c protein is working properly, it helps the cells shuttle heme and its building blocks and get them where they need to go in the cell. When this gene is knocked out or mutated, heme can build up in the parasite cells, making them more likely to activate the artemisinin “bomb.”
Another gene, when mutated, made the parasites less sensitive to artemisinin. The gene, called DegP2, encodes a protein that plays a role in heme metabolism, so when it was mutated, less heme was available in the cells to activate the drug.
This knowledge provides useful insights for treatment methods, said Lourido. For example, healthcare providers should take into consideration the fact that heme is key in artemisinin’s action, and avoid combining the drug with other treatments that might lower the amount of heme in parasite cells. “Understanding how different pathways within the cell participate to render parasites susceptible to these antiparasitic drugs helps us better pair them with other compounds that are going to be synergistic and not work against our own goal of defeating parasites,” Lourido said.
Taking the edge off toxic treatments
Another application of fundamental pharmacokinetics research involves mitigating the harmful effects of drugs. Consider the chemotherapy drug methotrexate. Methotrexate was the very first targeted drug ever made. Developed more than 60 years ago by Dr. Sidney Farber, the drug acts by inhibiting a key molecule in the metabolic process that builds DNA and RNA, thereby interfering with basic functions of the cell and with DNA synthesis, repair and replication, helping to destroy cancerous cells in the body.
Methotrexate is still a widely used component of chemotherapy cocktails, especially for pediatric leukemia. In the human body, though, methotrexate is like a bull in a china shop. It is very effective at knocking back cancer, but the drug’s life-threatening side effects, including gut, liver, kidney and brain damage, often lead doctors to terminate their patients’ treatment early, or seriously compromise the survivors’ quality of life.
The drug was much-studied in the 70s, but research trailed off in the subsequent decades due to limits on the existing technologies. Nearly fifty years later, Naama Kanarek, then a postdoctoral researcher in the lab of former Whitehead Institute Member David Sabatini, decided to take a fundamental research approach to studying the effects of methotrexate, in hopes that she might discover some way to make the drug less toxic.
“We now have access to genetic tools that allow us to address long standing questions in a way that was not possible before,” said Kanarek, who now runs her own lab at Boston Children’s Hospital. “We can use a CRISPR screen, and instead of focusing on what is known, we can ask what is unknown about the drug. We can find new genes that are involved in the response of cells to the drug that were not found before simply because the tools were not there.”
The screen revealed one gene in particular that seemed to be playing a role in how sensitive cancer cells were to methotrexate, the researchers reported in Nature in July of 2018. The cells’ sensitivity is important, Kanarek said, because if the cells can be made more vulnerable to methotrexate, the duration of treatment or required dose could be reduced. “If we can reduce dose because we can improve efficacy, then we can reduce toxicity without compromising on the cure rates and that is good news to the patients,” Kanarek said.
The gene in question, called FTCD, encodes an enzyme involved in the breakdown of the amino acid histidine. When the gene was knocked out, cancer cells were less sensitive to methotrexate. When the pathway was boosted with the addition of extra histidine, cells became more sensitive.
Former Whitehead Institute Director Susan Lindquist, who passed away 2016, performed similar work on the natural product amphotericin B, a drug which is used to treat some fungal infections. The drug is especially useful because fungi have not yet developed a resistance to it, as they have with so many other treatments. But amphotericin B also has some serious drawbacks; it can cause kidney damage, heart failure, and other serious and even fatal side effects.
These side effects mostly happened because amphotericin B works by binding to a chemical group called a sterol. In fungi, it binds to molecules called ergosterols in the cell wall, destabilizing the cells. Unfortunately humans also have a prevalent sterol: cholesterol. When amphotericin B binds to cholesterol in human cell membranes, it can damage human cells.
Using chemical synthesis methods, Lindquist and colleagues at Whitehead Institute and elsewhere were able to tweak the structure of the drug to bind only to ergosterol molecules and not cholesterol, bypassing most of the harmful side effects.
Why fundamental research
Drug development is often an extremely targeted pursuit, but for Whitehead Institute scientists, their advances have mostly come from a simple curiosity about the cellular mechanisms. For example, Rick Young didn’t set out to study condensates, but an inquiry into the fundamentals of transcription led him in this entirely new direction.
Such fundamental research has the potential to branch in any number of different ways. “Fundamental science can lead in directions that you would not foresee,” said the Institute’s Associate Director of Intellectual Property Shoji Takahashi. Basic research into drug behavior is essential and can contribute to life-changing therapies down the line.