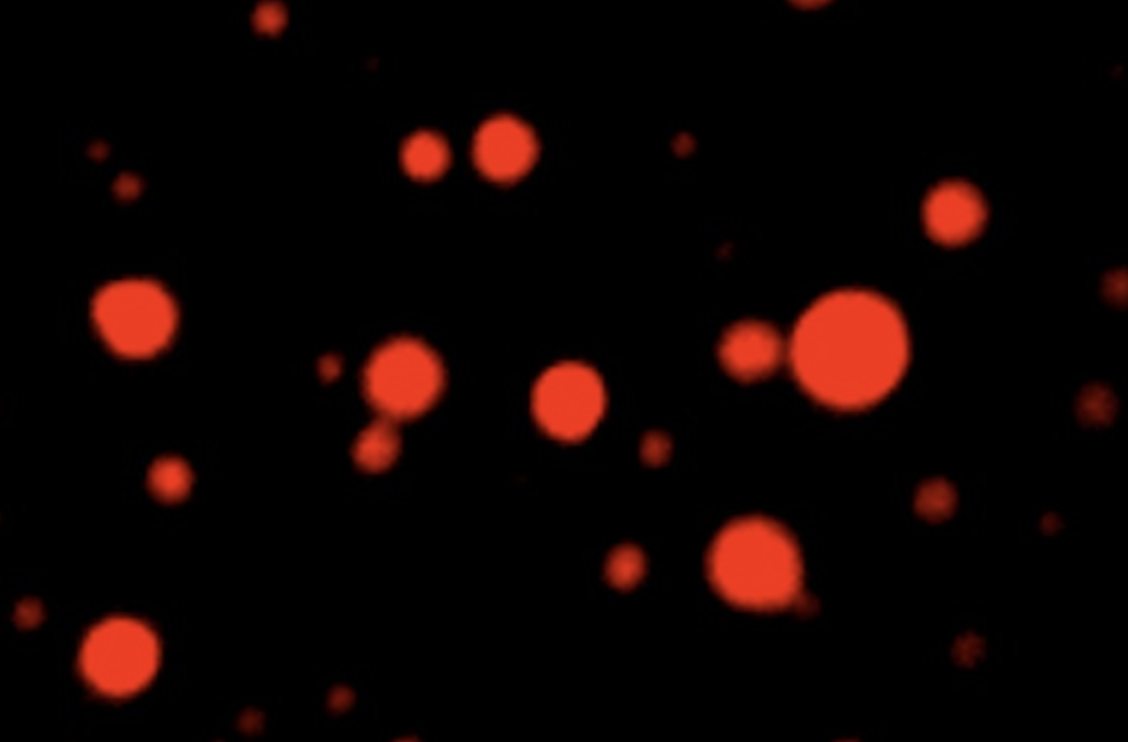
Whitehead Institute
June 17, 2020
In the watery inside of a cell, complex processes take place in tiny functional compartments called organelles. Energy-producing mitochondria are organelles, as is the frilly golgi apparatus, which helps to transport cellular materials. Both of these compartments are bound by thin membranes.
But in the past few years, research at Whitehead Institute and elsewhere has shown that there are other cellular organelles held together without a membrane. These organelles, called condensates, are tiny droplets which keep certain proteins close together amidst the chaos of the cell, allowing complex functions to take place within. “We know of about 20 types of condensate in the cell so far,” says Isaac Klein, a postdoc in Richard Young’s lab at Whitehead Institute and oncologist at the Dana-Farber Cancer Institute.
Now, in a paper published in Science on June 19, Klein and Ann Boija, another postdoc in Young’s lab, show the mechanism by which small molecules, including cancer drugs, are concentrated in these cellular droplets — a finding that could have implications for the development of new cancer therapeutics. If researchers could tailor a chemical to seek out and concentrate in one kind of droplet in particular, it might have a positive effect on the delivery efficiency of the drug. “We thought, maybe that’s an avenue by which we can improve cancer treatments and discover new ones,” says Klein.
“This [research] is part of a revolutionary new way of looking at the organization within cells,” says Phillip Sharp, a professor at the Massachusetts Institute of Technology’s Koch Institute for Integrative Cancer Research and a co-author on the study. “Cells are not little pools of soup, all mixed together. They are actually highly organized, compartmentalized units, and that organization is important in their function and in their diseases. We’ve just started to understand that, and this new paper is a really important step, using that insight, to understand how to potentially treat diseases differently.”
CONDENSATES AND DRUG DELIVERY
To explore how different properties of condensates inside the cell’s nucleus affected the delivery of cancer drugs, Boija and Klein selected a few example condensates to study. These included splicing speckles, which store cellular materials needed for RNA splicing, nucleoli, where ribosomes are formed, and a new kind of droplet Young’s lab discovered in 2018 called a transcriptional condensate. These new condensates bring together all the different proteins needed to successfully transcribe a gene.
The researchers created their own suite of four different fluorescently-labeled condensates by adding glowing tags to marker proteins specific to each kind of droplet. For example, transcriptional condensates are marked by the droplet-forming protein MED1, splicing speckles by a protein called SRSF2, and nucleoli by FIB1 and NPM1.
Now that they could tell individual droplets apart by their cellular purpose, the team, along with the help of Nathanael Gray, a chemical biologist at Harvard University and the Dana-Farber Cancer Institute, created fluorescent versions of clinically important drugs. The tested drugs included cisplatin and mitoxantrone, two anti-tumor medicines commonly used in chemotherapy. These therapeutics were the perfect test subjects, because they both target proteins that lie within nuclear condensates.
The researchers added the cancer drugs to a mixture containing various droplets (and only droplets, none of the actual drug targets), and found that the drugs sorted themselves into specific condensates. Mitoxantrone concentrated in condensates marked by MED1, FIB1 and NPM1, selectively avoiding the others. Cisplatin, too, showed a particular affinity for droplets held together by MED1.
“The big discovery with these in vitro studies is that a drug can concentrate within transcriptional condensate independent of its target,” Boija says. “We used to think that drugs come to the right place because their targets are there, but in our in vitro system, the target is not there. That’s really informative — it shows the drug is actually being concentrated in a different way than we thought.”
To understand why some drugs were drawn into transcriptional condensates, they screened a panel of chemically-modified dyes and found that the important part of many drugs — the part that led them to concentrate in transcriptional condensates — is the molecules’ aromatic ring structure. Aromatic rings are stable, ring-shaped groupings of carbon atoms. The aromatic ring in some drugs are thought to stack with rings in MED1’s amino acids, leading the drug to concentrate in transcriptional condensates.
Being able to tailor a drug to enter a certain condensate is a powerful tool for drug developers. “We found that if we add an aromatic group to a molecule, it becomes concentrated within the transcriptional condensate,” Boija says. “It’s that type of interaction that is important when we design new drugs to enter transcriptional condensates — and maybe we can improve existing drugs by modifying their structure. This will be very exciting to look into.”
WHERE DRUGS CONCENTRATE AFFECTS HOW WELL THEY FIGHT CANCER
In order for this tool to be practically useful in drug development, the researchers had to make sure that concentration in specific droplets would actually impact the drugs’ performance. Boija and Klein decided to test this using cisplatin, which is drawn to transcriptional condensates by MED1 and works to fight cancer by adding clunky platinum molecules to DNA strands. This damages tumor cells’ genetic material. When the researchers administered cisplatin to a mixture of different condensates, both in the test tube and in cells, the drug preferentially altered DNA that lay within transcriptional condensates.
This could explain why cisplatin and other platinum drugs are effective against so many diverse cancers, says Young, who is also a professor of biology at MIT; cancer-causing genes often carry regions of DNA called super enhancers, which are extremely active in transcription, leading to very large transcriptional condensates. “We now think the reason that drugs like cisplatin can work well in patients with diverse cancers is because they’re becoming selectively concentrated at the cancer-causing genes, where these large transcriptional condensates occur,” he said. “The effect is to have the drug home in on the gene that’s causing each cancer to be so deadly.”
A DRUG RESISTANCE MYSTERY, SOLVED
The new insights in condensate behavior also provided some answers to another question in cancer research: why people become immune to the breast cancer drug tamoxifen.Tamoxifen works by attaching itself to estrogen receptors in the cancer cells, preventing them from getting the hormones they need to grow and eventually slowing or stopping the formation of new cancer cells altogether. The drug is one of the most effective treatments for the disease, reducing recurrence rates for ER+ breast cancers by around 50%.
Unfortunately, many patients quickly develop a resistance to tamoxifen — sometimes as soon as a few months after they start taking it. This happens in a variety of ways — for example, sometimes the cancer cells will mutate to be able to kick the tamoxifen out of the cells, or simply produce fewer estrogen receptors for the drug to bind. One form of resistance was associated with an overproduction of the protein MED1, but scientists didn’t know why.
With their newfound knowledge of how a drug’s activity is affected by where it concentrates, Boija and Klein had a hypothesis: the extra MED1 might increase the size of the droplets, effectively diluting the concentration of tamoxifen and making it more difficult for the drug to bind its targets. When they tested this in the laboratory, the team found that more MED1 did indeed cause larger droplets, leading to lower concentrations of tamoxifen.
A NEW TOOLSET FOR DRUG DESIGNERS
The ability to better understand the behavior of drugs in cancer cells — how they concentrate, and why the cancer could become resistant to them — may provide drug developers with a new arsenal of tools to craft efficient therapeutics. “This study suggests that we should be exploring whether we can design or isolate drugs that are concentrated in a given condensate, and to understand how existing drugs are concentrated in the cell,” says Phil Sharp. “I think this is really important for drug development — and I think [figuring it out] is going to be fun.”