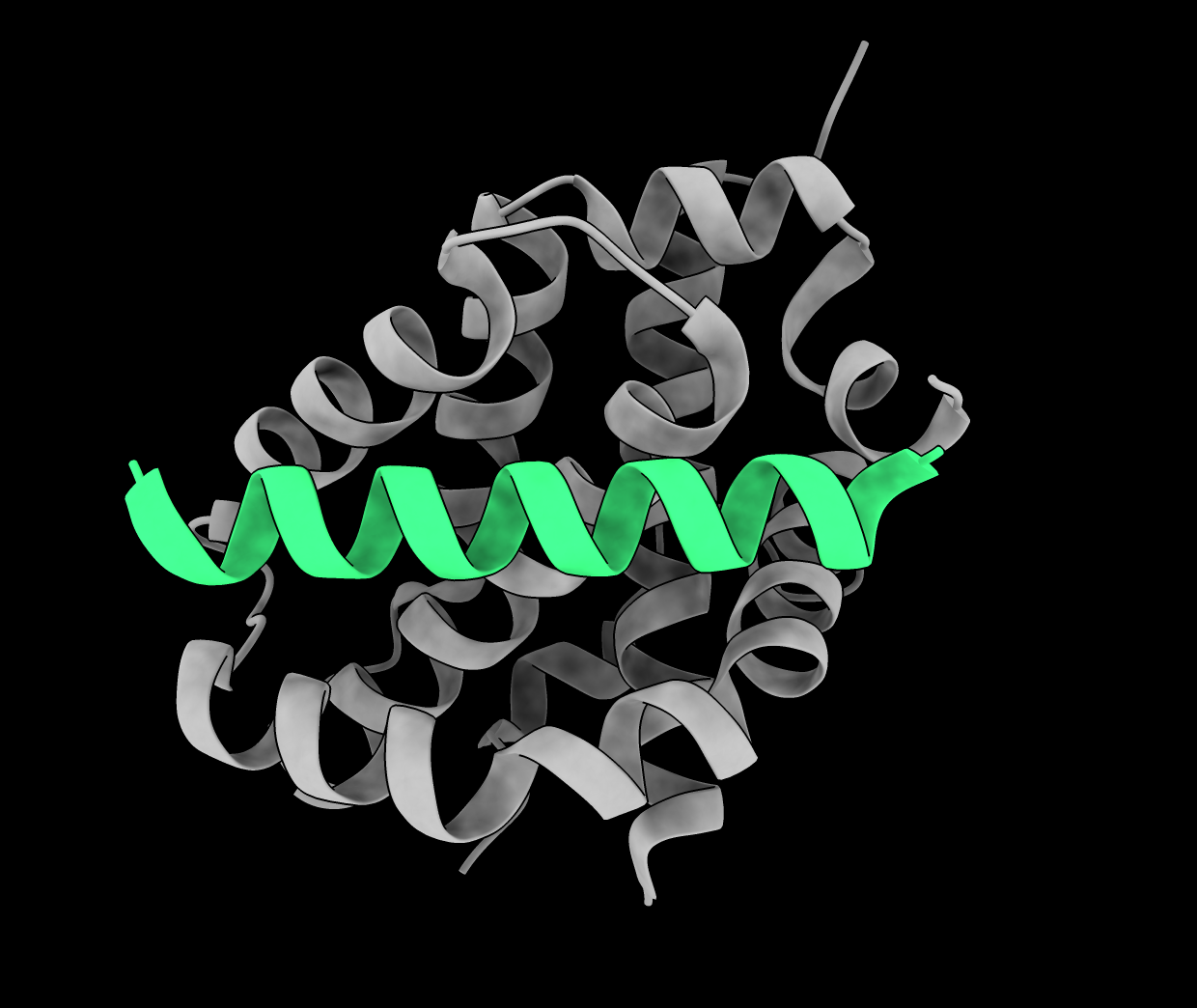
Biophysical characteristics such as peptide binding affinity and kinetics do not determine cell death function
Lillian Eden | Department of Biology
May 9, 2023
Billions of times a day, every day of our lives, cells receive signals to initiate the process of cell death. This strategic cell death, also called apoptosis, is one of the tools multicellular organisms use to maintain tissues and regulate immune responses: damaged, old, or superfluous cells are given the green light to, as it were, turn out the lights for the last time.
Programmed cell death is both extremely powerful and extremely regulated: for example, the careful culling of cells between our digits during embryonic development reveals fingers and toes. When programmed cell death goes awry, however, it can have serious consequences. Cells left unchecked can divide unstoppably and aggressively, leading to cancer. Dysregulated apoptotic pathways have also been implicated in neurodegenerative diseases like Alzheimer’s, where unrestrained cell death may play a part in the severity of the disease.
MIT Professor H. Robert Horvitz ‘68 shared a Nobel prize in 2002 for his foundational research on the genetics of programmed cell death and organ development in the nematode, a microscopic roundworm. Horvitz discovered that ced-9, a key gene in programmed cell death in nematodes, was similar in structure and function to the human gene bcl-2.
Targeting members of the BCL-2 protein family has already shown promise in the fight against cancer. For example, approved by the FDA in 2016, the oral drug Venetoclax is a BCL-2 inhibitor used to treat certain types of leukemia.
In a study published online Jan. 26 in Structure, Fiona Aguilar PhD ‘22 (Keating lab) and collaborators focused on a member of the BCL-2 protein family called BAK. When it is active, BAK promotes mitochondrial outer membrane disruption, leading to cell death, and is therefore referred to as a pro-apoptotic protein. But precisely how BAK becomes activated – or inhibited – is unknown.
“A greater understanding of BAK activation is interesting both from a fundamental biochemical and biophysical perspective as well as from the more translational one of BAK as a potential therapeutic target,” says lead author Fiona Aguilar.
BAK exists in two different forms: an inactive monomer and an active oligomer. A few activators of BAK (BIM, truncated BID, and PUMA) have already been identified and these proteins bind directly to BAK, leading to the model that binding of activators trigger changes in protein shape that allow BAK to transition from the inactive to active forms. To further explore this idea, Aguilar identified and characterized a number of other peptides that bind to and regulate BAK. To identify new peptide binders, the team used cell-surface display screening and computational protein design methods, including techniques developed by Keating lab alum Gevorg Grigoryan– dTERMen and TERMify – that use protein structural data to generate new protein sequences likely to bind a protein of interest.
In total, Aguilar et al. discovered 10 diverse new peptide binders of BAK that regulate its function.
Interestingly, some of the BAK-binding peptides inhibited activation rather than promoting it. Aguilar et al. found that inhibitors and activators of BAK shared many characteristics including structure as well as binding affinity and kinetics – the strength and rate that binders associate with and dissociate from BAK.
Newly identified activators had sequences both dissimilar from one another and from the previously known BAK activators BIM, truncated BID, and PUMA. The similarity of the sequence was not necessarily a good indicator of activation or inhibition. For example, an inhibitor and an activator differed by just two amino acids.
Aguilar and colleagues solved the crystal structures of two inhibitor-BAK complexes and one activator-BAK complex and found that the activator interacted with BAK with similar geometry as the two inhibitors. Also, the two inhibitors have only about 40% sequence identity, but bind very similarly to BAK.
Amy Keating, the senior author on the study, says “Fiona was tireless in identifying new peptides, testing their interactions with BAK, determining their functions, and solving structures to look for differences between activators and inhibitors. We were surprised that peptides with such different behaviors shared such common interaction properties.”
Although the puzzle is not yet solved, Aguilar believes the “transition state” between inactive and active forms of BAK is key.
“We think of activators as peptides that preferentially bind to the BAK transition state, whereas inhibitors are those that preferentially bind to the monomeric state,” Aguilar says. “Overall, we should be thinking more about the transition state, what steps are necessary to reach the transition state, and how to target the transition state.”
This study also added two sequences in the human proteome – BNIP5 and PXT1 – to the repertoire of known BAK binders. Not much is known about these sequences, Aguilar says, but the fact that they activate BAK could indicate that they may play a role in apoptotic pathways that have not yet been determined.
“The finding is something that people in the field are pretty excited about,” Aguilar says.
Ultimately, work remains to establish what characteristics of the binders determine their function, and how binding to BAK triggers the conformational changes that activate or inhibit this complex protein.
“It’s still unclear what it is about these sequences that trigger the allosteric network leading to BAK activation, but at least for now we can rule out the hypothesis that binding mode, affinity, and kinetics fully determine how this occurs,” Aguilar says.
Aguilar suggests that it will be interesting also to explore how these peptides interact with BAX, another pro-apoptotic protein in the BCL-2 family that is both structurally and functionally similar to BAK.
Fiona Aguilar is lead author and Amy Keating is senior author; Bob Grant and graduate students Sebastian Swanson, Dia Ghose, and Bonnie Su contributed. Collaborators Stacey Yu and Kristopher Sarosiek, from the Harvard T.H. Chan School of Public Health, helped with cell-based experiments. The research was funded by a National Institute of General Medical Sciences award, the MIT School of Science Fellowship in Cancer Research award, the John W. Jarve (1978) Seed Fund for Science Innovation (MIT) award, an award from the National Cancer Institute, a National Institute of Diabetes and Digestive and Kidney Diseases award, and Alex’s Lemonade Stand Foundation for Childhood Cancers award.