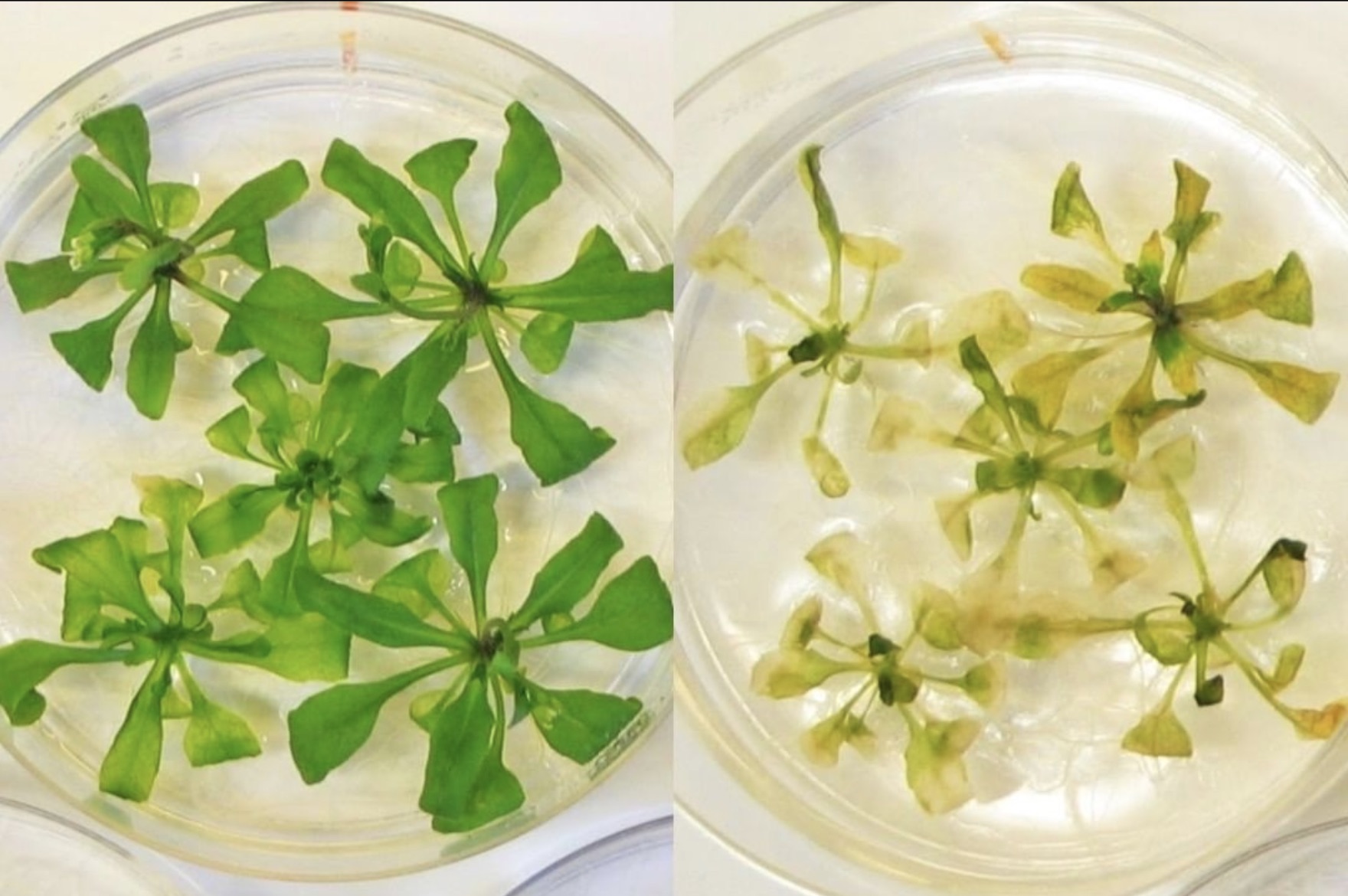
Nicole Giese Rura | Whitehead Institute
November 25, 2019
Salicylic acid, which may be best known as a treatment for skin conditions such as acne and warts and in its modified form as aspirin, is a critical plant hormone involved in growth and development as well as regulating plants’ immune defenses. Unable to move and evade physical damage or attacks by bacteria and other pathogens, plants respond to these assaults through the biosynthesis of salicylic acid, which in turn controls cascades of other defense responses. Consequently, control of salicylic acid production in agricultural plants could boost crops’ resilience to pathogens and insects, thereby reducing the overuse of potentially toxic pesticides that can lead to pathogen resistance. Yet scientists have been missing a key tool necessary for manipulating salicylic acid levels in plants: a full description of the pathway necessary to synthesize the hormone. Now Whitehead Institute Member Jing-Ke Weng, along with Weng lab postdoc Michael Torrens-Spence, have uncovered the last missing steps in the Arabidopsis plant’s salicylic acid pathway and solved a puzzle that has dogged Weng and his field for decades.
The quest to define the salicylic acid biosynthesis pathway started about 50 years ago when researchers determined that salicylic acid is principally formed downstream from a ubiquitous compound called chorismate. In 2001 another step was resolved: Chorismate is converted to isochorismate before eventually becoming salicylic acid. Encouraged by this progress, many in the fields of plant biology and biochemistry thought that the rest of the biosynthesis pathway in plants would be quickly defined by looking for enzymes similar to those that comprise the bacterial version of the pathway, rather an almost two decade-long drought in discoveries followed instead.
Weng and Torrens-Spence tried a different tack using genetic and biochemical methods to break the dry spell in the identification of the pathway’s missing links. Their work is described online this week in the journal Molecular Plant. From previous research, Torrens-Spence knew that the enzymes encoded by two genes – PBS3 and EPS1 – play roles in salicylic acid accumulation after pathogen attacks. In order to determine the role of these enzymes in salicylic acid biosynthesis pathway, Torrens-Spence generated plants lacking in S3H and DMR6, two genes known to breakdown salicylic acid and keep its production in check. With those genes disrupted, plants overproduce salicylic acid to an extreme extent, resulting in a severely stunted growth and other physical traits associated with surplus salicylic acid. Using these transgenic plants, Torrens-Spence had a model in which he could see if a particular gene affects salicylic acid production: If Torrens-Spence mutates genes responsible for salicylic acid biosynthesis, salicylic acid production should be abolished along with the associated visible plant characteristics. Mutations in PBS3 and EPS1 did just that – they rescued the stunted phenotypes associated with salicylic acid overproduction, and the plants accumulated less salicylic acid in their leaves than plants without the PBS3 or EPS1 mutations.
Next Torrens-Spence analyzed and compared the metabolites – the compounds created by cellular processes – in the leaves of plants without mutations and plants with PBS3 or EPS1 mutations. The results identified the probable products of the PBS3 protein’s enzymatic activity and also determined that the EPS1 protein likely acts downstream of PBS3. In order to confirm PBS3 and EPS1’s roles in salicylic acid biosynthesis, Torrens-Spence recreated the pathway in the test tube and in a relative of the tobacco plant. In both models, the reconstructed pathway efficiently converts isochorismate into salicylic acid. Interestingly, Torrens-Spence found that the intermediate produced by PBS3 could be spontaneously converted to salicylic acid in plants, but EPS1 greatly increased this step’s efficiency.
A recent evolutionary study indicates that PBS3 and variations of this gene are found throughout flowering plants, and Torrens-Spence’s work uncovered that PBS3 is an essential enzyme in the production of salicylic acid likely across all flowering plants as well. EPS1 is found only within the mustard family, which includes broccoli, Brussel sprouts, and turnips. According to Torrens-Spence and Weng, other enzymes may fulfill a role similar in plants that lack EPS1. Though the EPS1 aspect of the biosynthesis pathway described by Torrens-Spence and Weng are specific to Arabidopsis, their work provides a roadmap that researchers could follow to explore salicylic acid production in other organisms.
Weng, who has been trying to solve salicylic acid’s biosynthesis pathway in plants since he was in graduate school, says that he’s proud to have finally identified the remaining steps in Arabidopsis. With the complete salicylic acid biosynthesis pathway in Arabidopsis now known, agricultural scientists can use it to try to precisely manipulate salicylic acid’s immunological benefits in crop plants without the stunted growth associated with its excessive production.
This work was supported by the Pew Scholar Program in the Biomedical Sciences, the Searle Scholars Program, and the National Science Foundation (CHE-1709616).
Written by Nicole Giese Rura
***
Jing-Ke Weng’s primary affiliation is with Whitehead Institute for Biomedical Research, where his laboratory is located and all his research is conducted. He is also an associate professor of biology at Massachusetts Institute of Technology.
***
Citation:
“PBS3 and EPS1 complete salicylic acid biosynthesis from isochorismate in Arabidopsis”
Molecular Plant, online November 21, 2019 [online] DOI:10.1016/j.molp.2019.11.005
Michael P. Torrens-Spence (1), Anastassia Bobokalonova(1,2), Valentina Carballo(1), Christopher M. Glinkerman(1), Tomáš Pluskal(1), Amber Shen(1,2), and Jing-Ke Weng(1,2)
1. Whitehead Institute, Cambridge, MA 02142, USA
2. Department of Biology, Massachusetts Institute of Technology, Cambridge, MA 02139, USA