Author: Raleigh McElvery
PhD students Maureen Buckley and Krista Pullen discuss the role of sex hormones in infectious diseases, with a focus on HIV/AIDS and COVID-19.
June 8, 2022
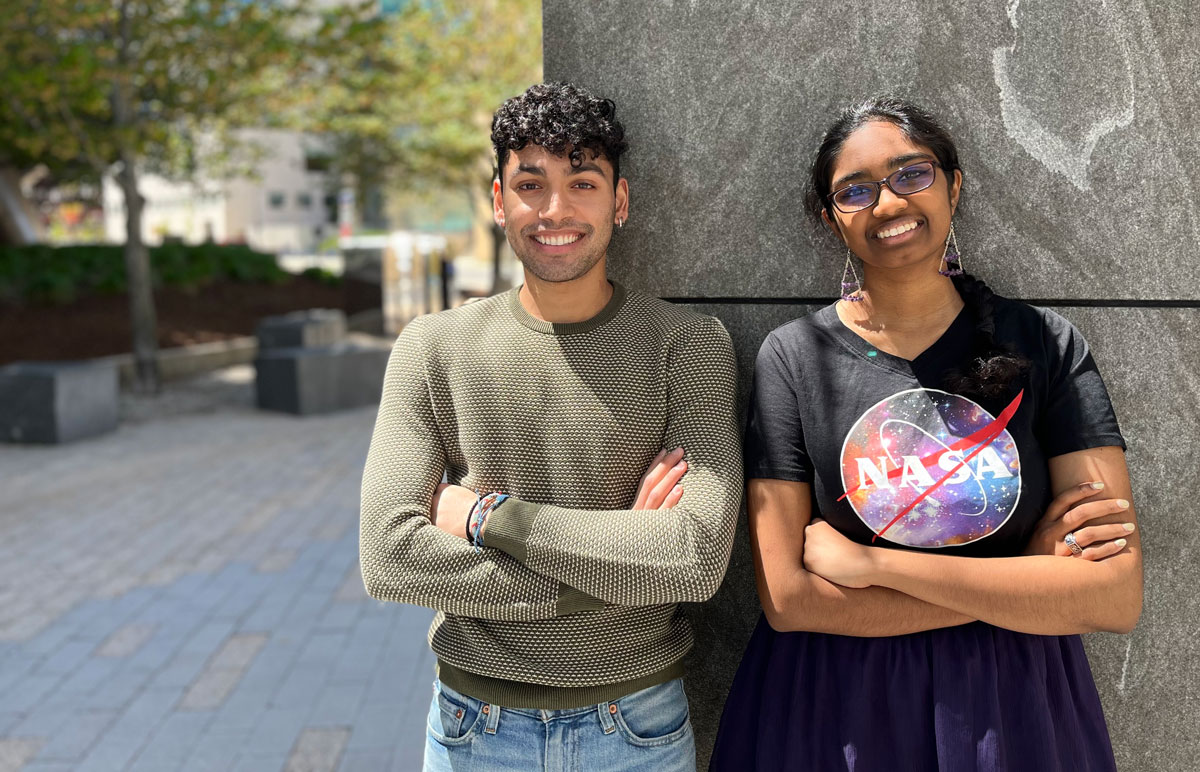
In a whirlwind team project, undergraduates Aniket Dehadrai SB ’22 and Brindha Rathinasabapathi SB ’24 of the Boyer lab pioneered a new method to study how hearts are built.
Celina Zhao
May 23, 2022
Can’t miss a beat
The lab was bustling with activity, with everyone working together on a team project comprised of many moving parts. Once one person finished a step of the experiment, it was whisked off to the next person. There was no time to lose.
During MIT’s Independent Activities Period (IAP) in January of 2022, several members of the Boyer Lab were hard at work — among them, Aniket Dehadrai, a junior studying Course 5-7 (Chemistry and Biology), and Brindha Rathinasabapathi, a sophomore studying Course 7 (Biology). Fueled with coffee every morning from the lab’s handy Keurig, the team was on a time crunch.
Working alongside Dehadrai and Rathinasabapathi were research scientist Vera Koledova, lab manager Kirsten Schneider, and fellow undergraduate researcher Caroline Zhang. They had a hard deadline at the end of the month to finish the project: studying how the absence of a certain protein affects the growth of cardiomyocytes, the cells responsible for pumping blood around the heart.
The Boyer lab — headed by Professor Laurie Boyer, the “Queen of Hearts” — specializes in heart cells. The lab is particularly interested in one intriguing question: Is it possible to heal the heart? Injuries like heart attacks often cause permanent damage that can eventually lead to heart failure. Scientists have found that at birth, injured heart cells are able to repair or replace themselves after such an event. However, that ability shuts off just a few days post-birth. Afterwards, heart cells, once damaged, are unfixable.
But what if adult cardiomyocytes could regain the ability to repair themselves, and thus repair trauma in heart tissue? The Boyer lab is intrigued by this possibility. But in order to answer that question, they must start from ground zero: learning how cardiomyocytes themselves develop.
The operation
Dehadrai, Rathinasabapathi, and the rest of the team were studying one part of that puzzle — the role histones play in cardiomyocyte growth. Histones are proteins that act as spools for DNA to wind around. DNA is extremely long, so histones help fit all this genetic information into the tiny space of a nucleus.
There are many types of histones (called “variants”), each of which has a unique effect on how DNA is wrapped. The tighter the DNA is packed, the more difficult it is for proteins to access the DNA — all of which affects how genes are expressed. As a result, each variant has a unique effect on how certain genes are regulated.
For the IAP project, the Boyer lab’s team focused on one histone variant called H2AZ.1. Prior studies have shown that H2AZ.1 is essential in most organisms, particularly when it comes to gene expression in stem cells. Stem cells are cells that essentially begin as blank slates, with the ability to form the many different cell types in the body. But through a differentiation process, they develop specific identities: skin, brain, or heart, to name a few.
By the end of the four weeks, the team planned to create and streamline a completely new process to “knock out,” or entirely remove, H2AZ.1 by degrading it during cardiomyocyte differentiation — the process where stem cells become specialized heart cells. Building this procedure to remove H2AZ.1 could later help identify what role H2AZ.1 plays in cardiomyocyte differentiation, a key step in both heart development and regeneration.
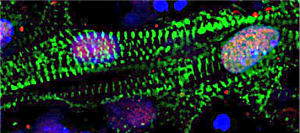
To begin creating the knockout procedure, the team started by culturing stem cells from a cell line specifically developed by the Boyer lab to study the H2AZ.1 histone. The goal was to see if removing H2AZ.1 would have a visible effect on how stem cells eventually become mature cardiomyocytes.
The amount of careful planning and execution to do in just one month — simply running through one full differentiation cycle took 15 days at a time — meant working together as a team was critical. “There was one late night with all five people in the lab, doing this giant experiment as well as we could without mixing up the different variables in play,” Rathinasabapathi says. “It was really critical for us to look over each other’s shoulders and double check each other.”
In all, the team tested out 10 different variations of a method to optimize the experimental procedure. Despite the time crunch, they succeeded in pioneering a procedure to efficiently remove H2AZ.1 during cardiac differentiation. It turns out that H2AZ.1 does, in fact, have a functional impact on heart cells.
Without H2AZ.1, the beating rate of mature cardiomyocytes was notably different, changing from rhythmic to arrhythmic. The research team also found varying levels of maturity in the cells, suggesting that the progression through the differentiation process was also changed.
All of this suggests that H2AZ.1 has a significant influence in gene regulation, which they plan to continue studying in greater detail in the future.
“We’re breaking new ground,” Dehadrai says. “And importantly, it’s a great framework for future work in this field.”
With the procedure the team developed, the lab is now able to ask and answer more questions. For one, they can zoom in on certain parts of cardiomyocyte differentiation to see when H2AZ.1 has the greatest impact on gene expression. They can also use this procedure as a model to study how other histone variants affect heart cell growth. Ultimately, they can begin piecing together how histones, their effect on gene regulation, and cardiomyocyte development unite to build the heart.
“The better we can understand how heart cell development works, the better we can understand heart development, injury, and response — all of which have a lot of different implications in the medical field,” Rathinasabapathi says.
Following their hearts
The two credit the cohesiveness of the team as a big part of their success. “Brindha is really responsible, helpful, and willing to put in the hours,” Dehadrai says . “You can’t take stuff like that for granted.”
“Ani is just as dependable, and I’ve learned a lot from him as a senior with a lot of experience in the lab,” Rathinasabapathi says.
Another strength of the team was their ability to draw upon many different academic areas: a hallmark of the Boyer lab, which is known for its interdisciplinary approach to heart research. Members come from all sorts of backgrounds: biology, chemistry, biological engineering, mechanical engineering, and more. Research in the lab also spans a wide expanse, from uncovering the secrets of heart regeneration to building better microscopy techniques to study the heart. In fact, that was one of the reasons why Dehadrai initially chose to join the lab. “Here, there’s people who pretty much know how to do everything,” he says.
Although the IAP project has concluded, both Dehadrai and Rathinasabapathi are committed to continuing their passion for medical research. Dehadrai, who is graduating in the spring, is planning to take a gap year to work on clinical research projects before applying to medical school.
Rathinasabapathi, on the other hand, still has two years at MIT. She plans to stay in the Boyer Lab and is eager to take more advanced courses in the Department of Biology. “I’m impatient — I wish I already had the solid foundation to attack the research at different angles and come up with more cool new things,” she says. “There’s just so much more that I want to know.”
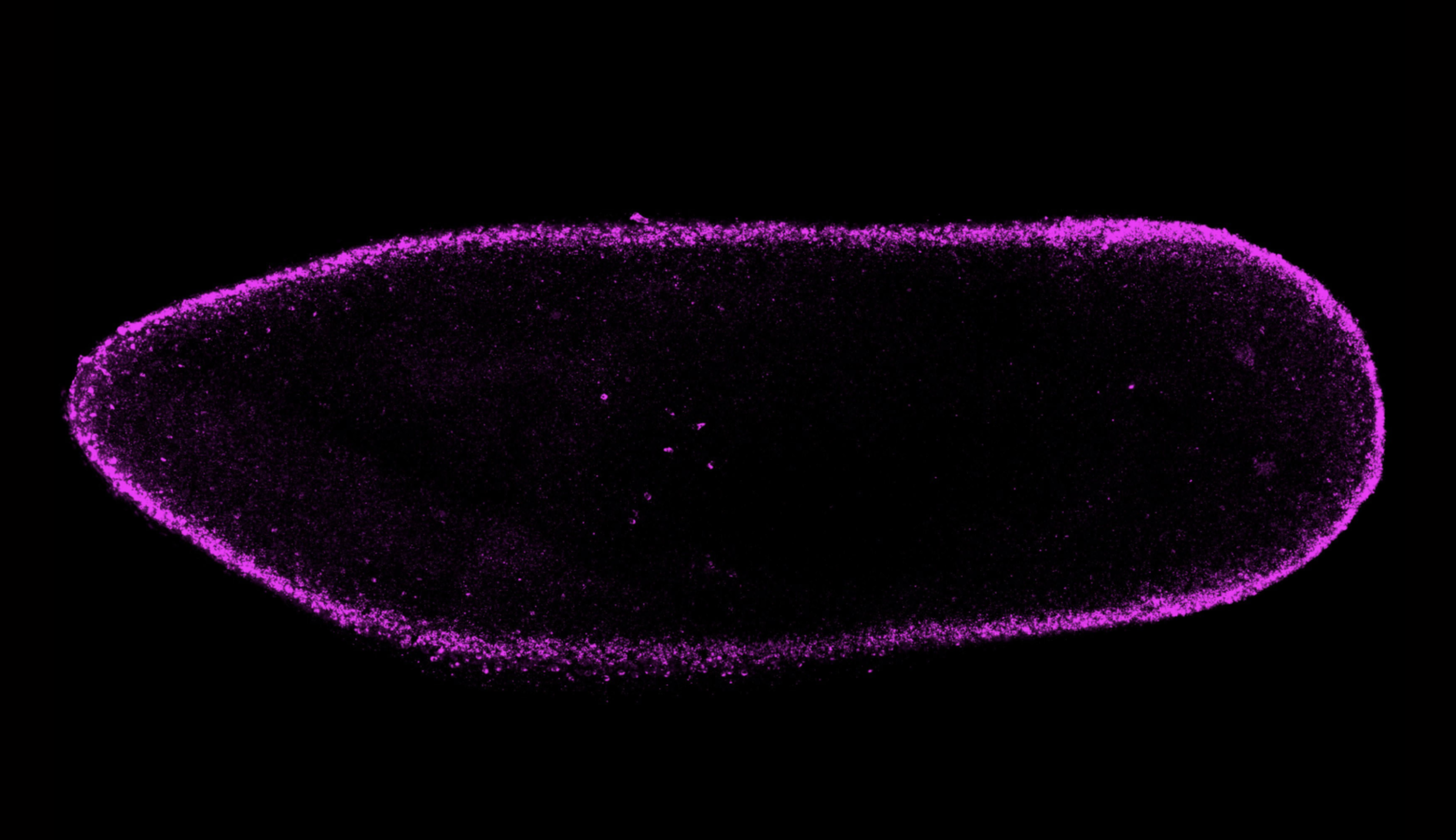
Greta Friar | Whitehead Institute
May 18, 2022
When animals experience a large injury, such as the loss of a limb, the body immediately begins a wound healing response that includes sealing the wound site and repairing local damage. In many animals, including humans, when the local wound site is taken care of, this response ends. However, in some animals, the initial wound response soon transitions into another stage of healing: regeneration, regrowing the parts that were lost.
Whitehead Institute Member Peter Reddien, also a professor of biology at MIT and a Howard Hughes Medical Investigator (HHMI), has long studied a flatworm known as the planarian (Schmidtea mediterranea), capable of regrowing any part of its body, to understand the mechanisms underlying regeneration. New research from staff scientist M. Lucila Scimone, graduate students Jennifer Cloutier and Chloe Maybrun, and Reddien identifies a previously undescribed gene, equinox, as playing a key role in initiating the transition from the initial wound healing stage into the regeneration stage in planarians. The work, published in Nature Communications on May 18, also reveals an important role for the wound epidermis, the skin that grows to cover a wound site, in initiating regeneration. Discovering what enables animals like planarians to regrow lost body parts can inform the field of regenerative medicine, which seeks to understand the limits of wound healing in humans and to improve our capacity for recovery and regeneration.
“The more we understand about the genes and mechanisms that play key roles in regeneration in animals that are capable of it, the better we may understand why humans lack that ability and, perhaps, the feasibility of future approaches to improve human wound healing,” says co-first author Scimone.
The case of the mystery gene
When the researchers began this project, they had no idea that it would lead them to identify a new gene that was crucial for regeneration. They originally set out to learn more about bmp4, a gene they had previously studied. BMP signaling, which includes bmp4, is involved in dorsal-ventral patterning, or the formation of the body around an axis between its top (dorsal) and bottom (ventral) sides. Previously, Reddien had found that bmp4 was necessary for regeneration after injuries to an animal’s side. Using new technologies that had not been around when they first studied the gene, the researchers now found that planarians without bmp4 failed to regenerate after large injuries anywhere on the body. This suggested a much more fundamental role for bmp4 in regeneration than the researchers expected, given that its main function relates to only one body axis. The researchers hypothesized that along with its role in dorsal-ventral patterning, bmp4 might help to activate an unknown gene that played some important, as yet unidentified role in regeneration. Bmp4 would therefore be necessary for regeneration because of its connection to this mystery gene.
The researchers started looking at genes regulated by bmp4 and found a promising candidate. They learned that bmp4 was needed to activate their mystery gene during the initial wound healing response, and that the mystery gene was crucial for wound healing to progress into regeneration after large injuries. When the gene was not activated, the steps that usually follow the initial wound healing response to prepare the body for regeneration would not occur. The wound would heal but the missing parts would never regrow, much like what would happen in a human. The researchers named the mystery gene equinox in honor of its appearance during a key transition period to move the body towards renewal.
“We know of a few genes that, when they are inactivated, the hallmarks of regeneration do not occur,” says co-first author Cloutier. “When equinox is not activated, we see an even more powerful inhibition of regeneration at an early phase. It appears to be required early on to allow for the other steps to proceed.”
Skin gets a starring role
The researchers found that equinox is expressed, or active in, wound epidermis, a skin tissue that is integral to regeneration after large injuries in a number of animals and yet had not been known to play a role in the signaling that initiates regeneration in planarians. After an injury, the wound epidermis covers and protects the wound site. As animals begin regeneration, the wound epidermis facilitates the formation of an outgrowth of cells called a blastema, in which the body produces the cell types it needs to replace the parts lost in the injury. Correspondingly, the researchers found that equinox is needed for regeneration in any injury that requires a blastema—essentially any large external injury where the replacement tissues grow out from the body.
Previously, the Reddien lab had found key genes required for regeneration expressed largely in muscle. Muscle in planarians maintains an active blueprint of the body, a network of positional genes that lets cells and tissues know where they are supposed to be. After an injury requiring regeneration, these positional genes rescale their body map near the wound site and guide new cells in building replacement tissues in the correct places. However, if equinox is not expressed, then the muscle tissue does not rescale its map. The body also fails to ramp up production of planarian stem cells or to begin differentiating stem cells into the cell types that were lost. Together, these findings flesh out the researchers’ understanding of the complete steps needed for regeneration to occur, revealing an early key role for wound epidermis, through its expression of equinox, in the signaling sequence that enables regrowth after an injury.
“There’s a cascade of events in which wound signaling activates, among other genes, equinox; equinox promotes wound-induced gene expression in muscle; and that promotes positional information resetting that can then lead to regeneration,” Reddien says. “What’s exciting about filling in this picture is that we’re identifying the key regulatory logic that can bring about regeneration.”
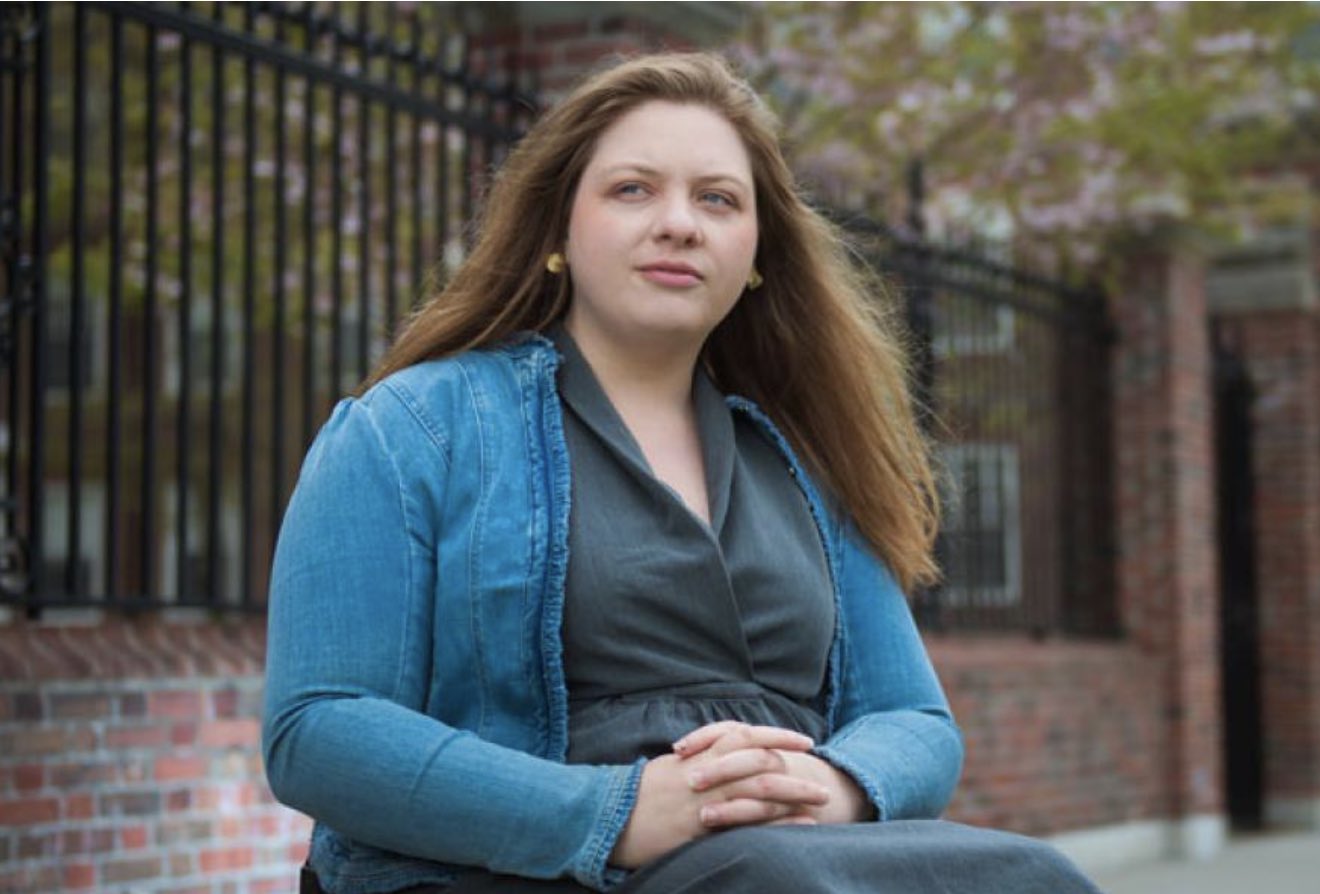
HMS grad Jennifer Cloutier has a habit of pushing limits
Christine Paul | Harvard Medical School News
May 17, 2022
When Jennifer Cloutier receives her MD from Harvard Medical School in May, it will be 12 years since she won a Canadian national waterskiing championship.
Although that feat alone is impressive, it’s even more extraordinary because the competition was designed for individuals with disabilities, and because of her lower-body paralysis, Cloutier, now 30, performed tricky slalom turns and acrobatics from a special seat bolted to her skis.
But then, pushing limits has been Cloutier’s signature style.
“In the 20-second period allowed for trick skiing, if you fall off the seat, your performance is over,” she said. “So, my goal was to always perform the hardest trick I could do without falling.”
Skiing triumphs were just the beginning of many of Cloutier’s achievements, demonstrating her refusal to be deterred by the spinal-cord injury she experienced at age 6 in a car accident, which also left her younger brother paralyzed.
Pushing limits
Cloutier was encouraged by her parents not to let her injury impede her future ambitions, and during the six months she was initially hospitalized after the accident, she gained firsthand appreciation of the marvels of rehabilitative medicine, which she says helped inspire her to become a doctor.
But childhood came first. At age 10, the Ottawa, Ontario, native also embraced alpine skiing, becoming a ski instructor during high school.
Then, turning to watersports, she competed internationally and became a volunteer administrator for SkiAbility Ottawa, a waterskiing organization for people with chronic illnesses and disabilities.
Winning medal after medal, Cloutier’s athletic successes and volunteer work with disabled people culminated in her being selected in 2011 to Canada’s Top 20 Under 20, a prestigious list published by Youth in Motion.
At the time, Cloutier was already at Harvard College, graduating with a bachelor’s in human developmental and regenerative biology in 2013, and serving as president of Women in Science at Harvard-Radcliffe from 2011 to 2013.
She says her early traumatic injury was pivotal in defining her research goal—to understand how tissues regenerate after they are damaged. HMS and the Massachusetts Institute of Technology (MIT) have given her a unique opportunity to pursue this goal.
Enrolled in the joint Harvard-MIT Program in Health Sciences and Technology (HST), which immerses students in rigorous interdisciplinary studies on both campuses, Cloutier will receive an MD in 2022 from HMS, complementing the PhD in biology she received from MIT in 2020.
Compressed into overlapping years, HST students on the MD track receive training to become physician-scientists. In addition to classroom studies on the HMS campus and clinical rotations at HMS-affiliated hospitals, they spend long hours in HMS or MIT laboratories, working with leading scientists on critical questions.
“As a physician-scientist, I am very interested in how organs and tissues re-form in adult organisms that are attempting to regenerate from injury,” Cloutier said.
She has studied the regenerative ability of a tiny planarian, or flatworm, named Schmidtea mediterranea.
For two centuries, this freshwater planarian has been a model organism for studying development and regeneration, because of its distinct anatomical features—eyes, gut, brain, central nervous system, and more—and its capacity to regenerate any missing body region, even the whole body, from minuscule body parts.
Working in the lab of Peter Reddien, professor and associate head of the MIT Department of Biology, Cloutier’s research has focused on planarian signaling pathways that recruit stem cells for regenerating tissues.
“Our research team is seeking to identify the genes and signals involved in initiating regeneration,” Cloutier said. “We are converging on a promising regulator that is expressed within hours of injury in the planarian wound epidermis. Such a discovery would offer key insights to the cellular signals that drive regeneration and could potentially lead someday to therapeutic strategies for better repair after injury,” she said.
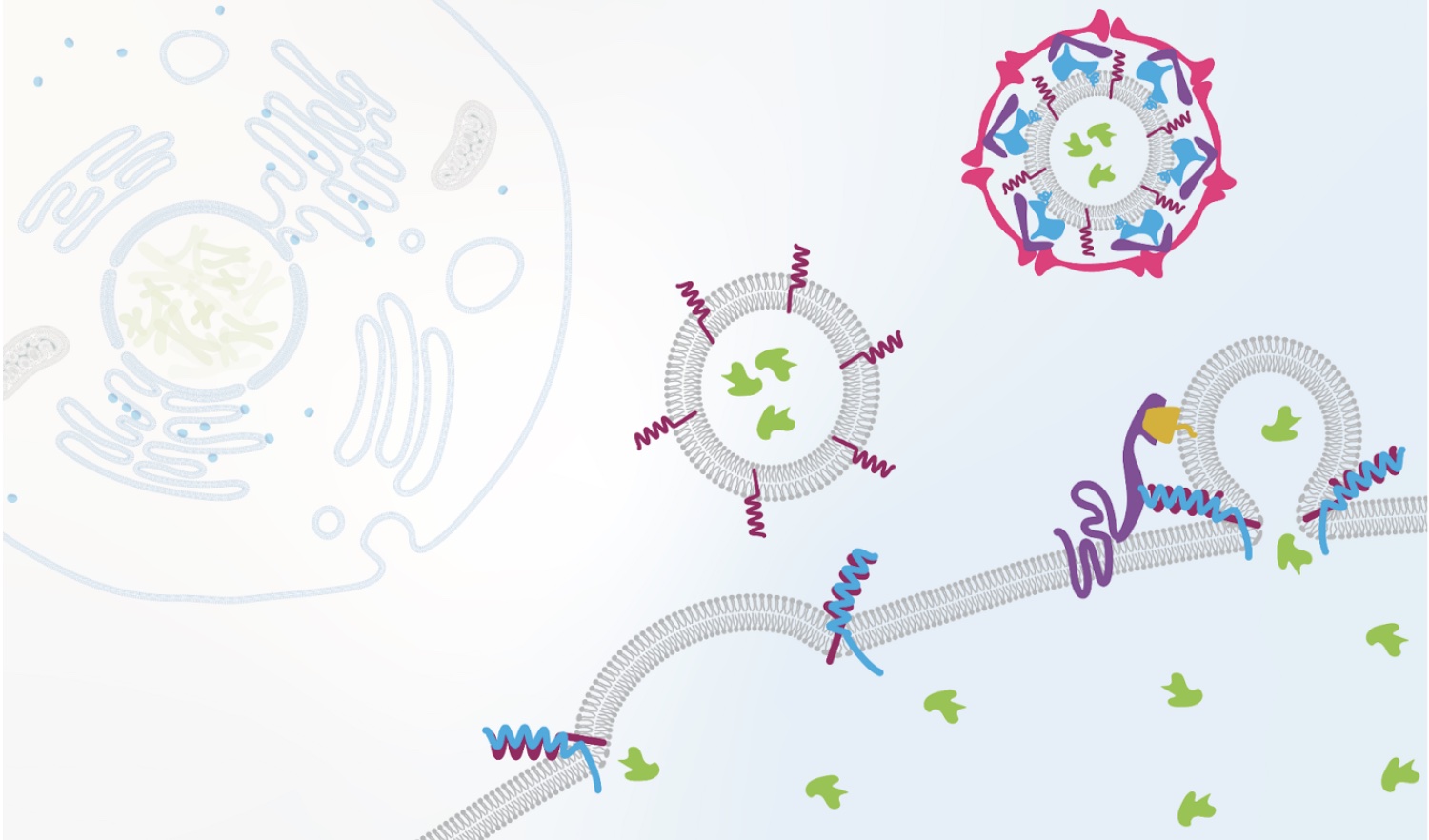
Producing multimedia for online courses involves lifelong learning
Darcy G. Gordon, Instructor of Blended and Online Learning Initiatives, MITx Digital Learning Scientist, Biology
May 11, 2022
As a biologist who has made my own figures for publications, I’ve always appreciated well-constructed, easy-to-understand, and scientifically accurate visualizations, but admittedly those appreciations were often fleeting and superficial. I did not fully realize the incredible detail and thought that are required for making effective scientific visualizations used for teaching. Once I joined the MITx Biology team and became the lead on making visual resources for our online course offerings (most notably 7.05x Biochemistry and the 7.06x Cell Biology series, parts 1, 2, and 3), I came to understand visual representations of biological phenomena in a new way.
From cellular morphology captured in the stunning microscopy images at the Koch Institute to banding patterns in polyacrylamide gel electrophoresis, biologists often make sense of structural and functional relationships through the use of visual tools, and an often implicit part of biology education includes teaching visual scientific literacy. The cognitive effort behind interpreting visual representations one-by-one, let alone those that transition between different levels of biological organization or incorporate different kinds of representations of the same concept, is not trivial. In an intensely visual science such as biology, how can I make the connections between representations and concepts more seamless and intuitive for learners?
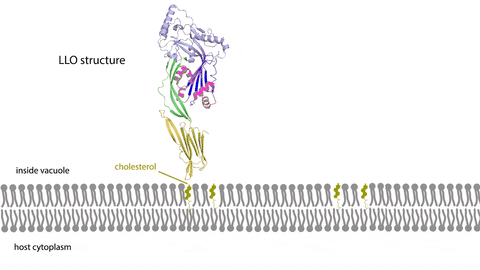
Learning multimedia tools and theory
I became engrossed in learning how to use new tools that would help me make abstract concepts come to life, like modeling 3D protein structures and animating cellular processes. As I learned the techniques and skills necessary to represent subcellular biological processes, I also studied the rich body of scholarly work that addresses what makes visual representations compelling and accessible. Applications of how we use this multimedia learning theory and other evidence-based practices in our courses is better summarized elsewhere, but I found these ideas immensely helpful in creating visual resources for worldwide learners.
Doing the work
Soon I was designing color palettes that maintained accessibility and cued learners to relationships between representations, simplifying schematics to convey essential processes and reduce extraneous details, and finding ways to emphasize important features through visually intuitive signaling. I spent hundreds of hours per course producing this content; drawing and animating biochemical reactions, editing the instructional videos captured during class, and revising the resulting media based on feedback.
Teaching as a way of understanding
Through the process of creating a couple thousand images, animations, and videos for MITx Biology, I have not only learned the technical and theoretical bases for multimedia design, but my understanding of core biological concepts has deepened. Updating my understanding of biological processes and conveying that understanding to others through multimedia, as well as sharing emerging best practices in teaching with technology, illustrates the iterative cycle of learning, doing, and teaching. Teaching others, whether it be about cellular signaling pathways or how to illustrate these pathways, has always been the best benchmark of where I am in my own learning journey.
A career in learning engineering bridges my academic training in biology, instructional expertise, and technological skills to make experiences in the classroom, whether it be virtual or in-person, meaningful for all. I also see this profession as an ongoing meditation on lifelong learning. Teaching worldwide learners about biology, or my peers about best practices in educational multimedia is a dynamic process. New information and technology change the way we understand how people learn and how cells function, and I must integrate these changes into my work to create engaging learning experiences for global audiences. Through interactions with the same material, albeit on different sides of a learning management system, I also enjoy a philosophical kinship with our MITx Biology participants. At some level, we share the belief that no matter where you are or what you do, there is always more to learn.
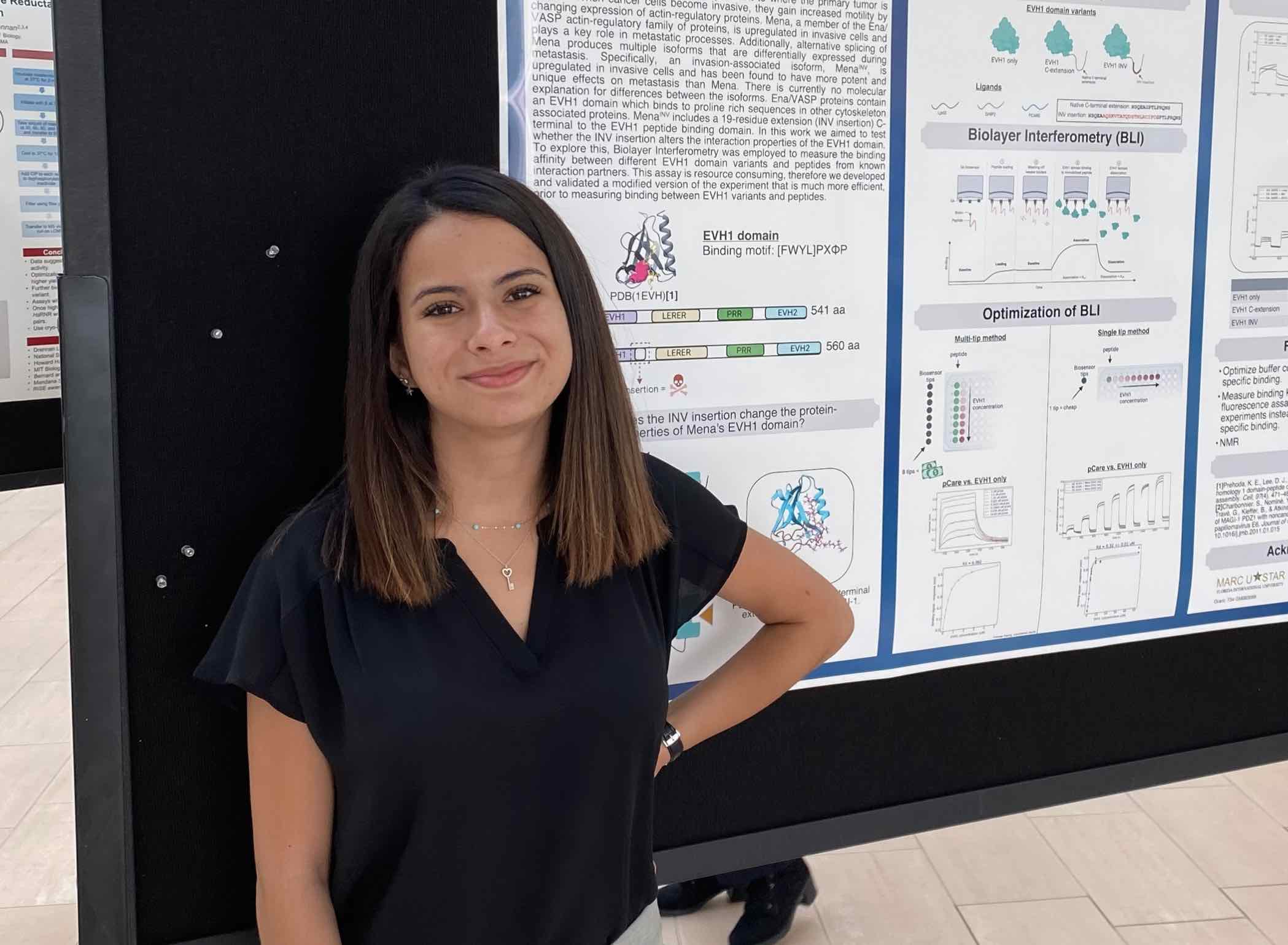
The MARC U*STAR program is equipping students with the skills to thrive in graduate school and land competitive jobs as researchers
Gisela Valencia | Florida International University
May 1, 2022
When Alejandra Ramos was 15 years old, she emigrated from Cuba to Miami with her family. She re-started her life, learned a new language and worked to understand the education system in her new home.
Today, she is set to graduate with a bachelor’s in biochemistry and top-notch research experience under her belt — including a summer internship at Massachusetts Institute of Technology (MIT), which extended into an additional semester-long stay as a visiting research student at the invitation of that university. She will be graduating in the spring of 2022 and will begin her Ph.D. in biology at MIT in the fall.
What’s the force behind her success? Her tenacity and her secret weapon: FIU’s MARC U*STAR Program, which hosted an event where Ramos met representatives from MIT — all of which led to the internship. Funded by the National Institutes of Health and acting as a branch of the countrywide initiative, the MARC U*STAR program at FIU, housed within the College of Arts, Sciences & Education, prepares undergraduate fellows for careers in research through mentorship, internships and lab work.
“The greatest mission of the program is to increase diversity in biomedical research,” says Amy Reid, the program’s coordinator. “The idea is to provide opportunities for traditionally underrepresented students, so they are prepared to apply for graduate programs. They also make a two-year commitment to participate in a research lab. This all makes them very competitive.”
Becoming researchers
The fellows earn hands-on experience at FIU labs — currently, students are researching topics including ovarian cancer, lung cancer, melanoma and the sleep cycles of mosquitoes. Students receive a stipend for their research work and a partial tuition waiver, as well as funding for travel to present their research at top national conferences, including the Annual Biomedical Research Conference for Minority Students (ABRCMS), where various students including Ramos have won awards for their work.
The students also participate in professional development workshops at FIU and enjoy a variety of networking opportunities to help them connect with future grad schools or employers — or simply to help them practice their professional communication skills.
“The FIU research mentors combined with the MARC program really give the students everything they need to be ready for graduate school,” Reid says. “Critical thinking, learning how to develop and test a research question and all those extra soft skills, like learning how to interview.”
The program requires students to participate in a summer internship at a university or organization outside FIU, giving them wide-reaching experiences and a greater competitive edge.
For biochemistry senior Celeste Marin, the summer internship — and the conferences — proved critical to discovering her dream job. While showcasing her research at an ABRCMS conference in 2020 (hosted virtually due to the pandemic), she met a representative from the renowned pharmaceutical company Eli Lilly. She was invited to interview for an internship at the company.
She succeeded and was offered the internship. Yearning to get a taste of the industry side of research, she accepted — and found her calling.
“During the internship, I realized I really want to work in the pharmaceutical industry,” says Marin, who also won an award for her research at the 2021 ABRCMS conference. “I’m very interested in drug discovery or therapy. To me, that sense of discovery, of problem-solving, of being a scientist, that’s what I find the most fun in terms of the work that I’ve done.”
She decided to apply to pharmacology and biochemistry Ph.D. programs and was accepted into various institutions including the University of Pennsylvania, University of North Carolina at Chapel Hill and the University of Florida. She is graduating this week, and in the fall, she will begin her Ph.D. in biochemistry at Duke University.
She says MARC U*STAR’s emphasis on networking was a gamechanger for her, one that allowed her to find her career.
“Networking was something I was iffy about,” she says. “To introduce myself and my research, to go out of my way to do that, it seemed difficult before. Now that I’ve done it a few times, it’s easier to go network, to have those moments of connection.”
Making a difference
Thanks to the program, many Panthers like Marin and Ramos have gained connections, found career paths and landed crucial opportunities.
“Honestly, the MARC program has changed my life,” Ramos says. “The program provided me with the tools, confidence and support that I needed to succeed. It gave me the confidence to apply to top graduate programs.”
She applied and was accepted into MIT, Harvard, Stanford, UC Berkeley, University of Central Florida, Johns Hopkins, Columbia and UC San Francisco.
“I would have never thought of applying to those programs if it wasn’t for the MARC program and [hearing about] previous fellows that have made it to those places,” Ramos says. “I wouldn’t be where I am today without the MARC program.”
Graduate student Maria Jose Santiago agrees. Just a few years ago, Santiago worked full-time at Home Depot. Today, she’s a doctoral student in biochemistry conducting pioneering research at FIU.
A Cuban immigrant who worked hard to support her family, Santiago sometimes felt her dream — earning a degree in biology — was impossible. But she worked hard, earned her associate’s degree at Miami-Dade, and came to FIU ready to succeed. She heard about the MARC U*STAR program, and it opened her eyes to the career that was truly, completely possible to achieve.
“When I joined MARC U*STAR, I felt that I was part of the scientific community,” recalls Santiago, who is now a McKnight Fellow and an FIU Transdisciplinary Biomolecular and Biomedical Sciences Fellow. “I felt like I belonged to something.”
Santiago says that one of the greatest aspects of the program is Reid — who mentors, helps and guides students every step of the way — and the support system of the MARC staff and students.
“It wasn’t just my principal investigator [researcher]. It was Amy [Reid],” Santiago says. “She was so sweet and caring. She helped me with applications, with everything. I didn’t feel alone.”
For her part, Reid says the students she helps are stars.
“Our students are extremely gifted,” she says. “I love watching the students come in with little experience and by the end of two years they are so confident and their skill level is unbelievable. They do presentations at the caliber of graduate students. They are really amazing. I’m always floored by their capabilities and what they achieve.”
If you’re wondering whether you should try the program, Santiago has a message for you:
“You should apply,” she says. “It’s going to be the best experience of your life. You’re going to have to work hard, but it’s totally worth it. You shouldn’t miss this opportunity.”
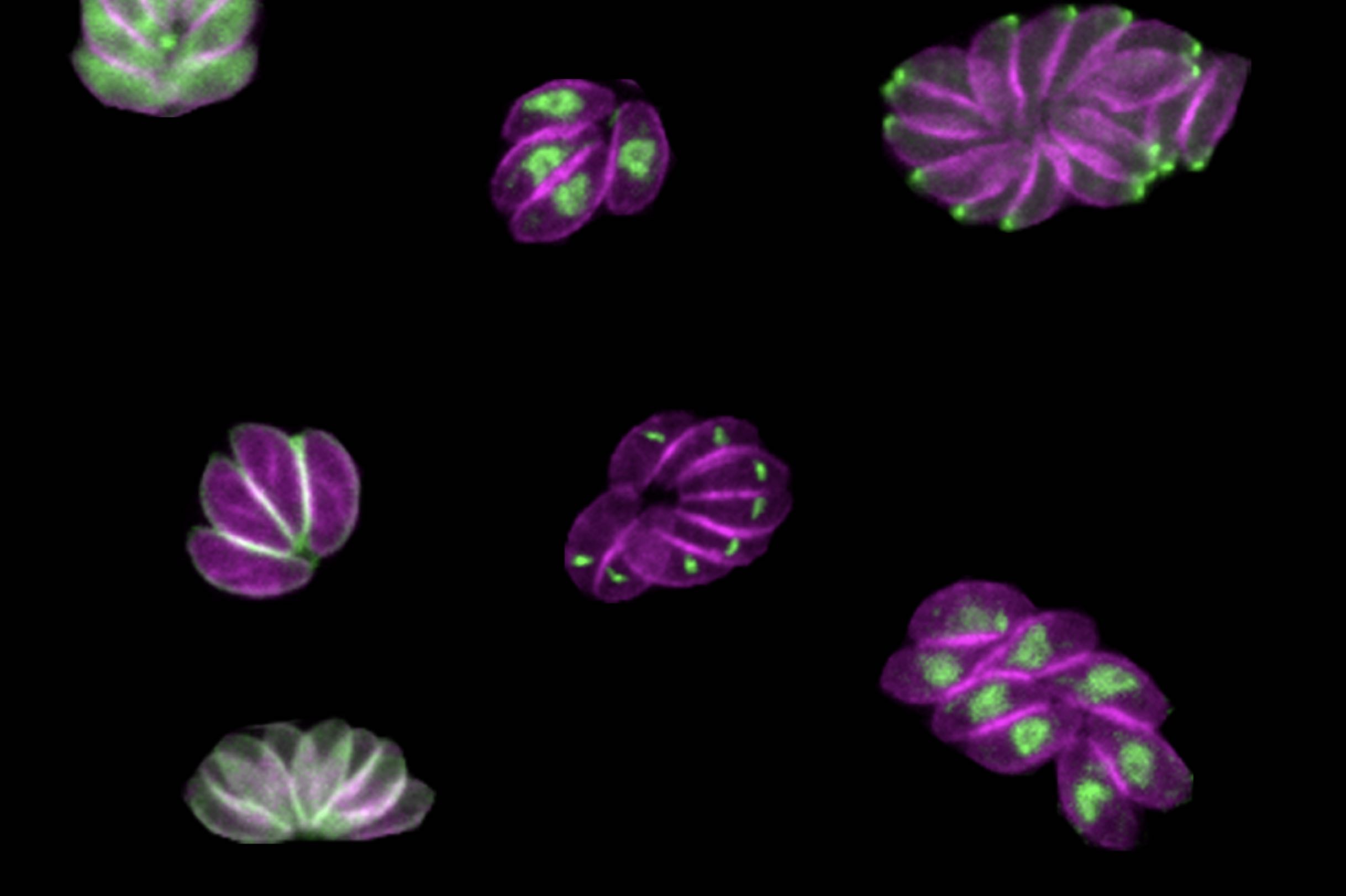
Eva Frederick | Whitehead Institute
April 28, 2022
Whitehead Institute Member Sebastian Lourido and his lab members study the parasite Toxoplasma gondii. The parasite causes the disease toxoplasmosis, which can be dangerous for pregnant or immunocompromised patients.
As the parasite evolved over millennia, its phylum (the Apicomplexan parasites) split off from other branches of life, which poses a challenge to researchers hoping to understand its genetics. “Toxoplasma is very highly diverged from the organisms that we typically study, like mice, yeast and [nematodes],” said Lourido lab researcher and Massachusetts Institute of Technology (MIT) graduate student Tyler Smith. “Our lab focuses a lot on developing toolkits to probe and study the genomes of these parasites.”
Now, in a paper published in the journal Nature Microbiology on April 28, Smith and colleagues describe a new method for determining the role of genes within the genome of the parasite. The method can be conducted by a single investigator, and goes a step beyond simply assessing whether or not a given gene is essential for survival. By inserting specific sequences — such as those encoding fluorescent markers or sequences that can turn a gene on and off — throughout the Toxoplasma genome, the method allows the researchers to visualize where an individual gene’s product resides within the parasites and identify when in the life cycle important genes became essential, providing more detailed information than a traditional CRISPR screen.
Although the method could theoretically be used with any gene family, Smith and Lourido decided to first focus on a family of proteins called kinases, the genetic code for which comprises around 150 of Toxoplasma’s 8,000 total genes.
“Kinases are interesting from a basic biology perspective because they are signaling hubs of basic biological processes,” said Smith, who is first author of the study. “From a more translational perspective, kinases are really common drug targets. We have a lot of inhibitors that work with kinases. For some cancers that are linked to specific kinases, the inhibitors can be chemotherapies.”
New dimensions of screening
Many CRISPR screens use gene editing technology to knock out genes throughout the genomes of a sample of cells, creating a population where every gene in the genome is mutated in at least one of the cells. Then, by looking at which mutations have detrimental effects on the cells, researchers can extrapolate which genes are essential for survival.
But the workings of a whole organism are infinitely more complicated than just survival or death, and researchers are often faced with a challenge when it comes to figuring out exactly what different gene products are doing in the cells. That’s why Smith and Lourido decided to design a method of screening for Toxoplasma genes that could provide more information about what the products of those genes do. “CRISPR screens can tell you which genes are important, but it doesn’t give you much information about why they’re important,” Smith said. “We were seeking to make a kind of platform that could look at other dimensions.”
Smith and Lourido used CRISPR technology to introduce small amounts of new DNA into the parasites’ genes that code for kinases. The new DNA included sequences encoding a fluorescent marker protein and sequences that could be used to manipulate gene expression levels.
After creating a population of parasites modified this way, the researchers then used imaging to determine where the fluorescently tagged proteins had ended up in the cells, and to observe what happened in the cells when the proteins were turned off. “Being able to see different cell division phenotypes — for instance parasites that either failed to replicate at all, or tried to replicate but would have some abnormalities — that gets us closer and allows us to generate hypotheses as to actually why these kinases are important, not just whether or not they are important,” Smith said.
The depletion of some proteins caused the parasites to die instantly, while others affected the parasites at a later point in their life cycles, so they would drop out of the population more slowly. “Cells with mutations in these kinases replicate fine, but a problem might arise when they need to leave their host cell and enter a new host cell later on down the line,” Smith said.
A “SPARK” of inspiration
After the screen, the researchers followed up on one of these kinases in particular, which they called SPARK (short for Store Potentiating/Activating Regulatory Kinase). Mutants depleted of SPARK died, but not until a later phase of the life cycle. Smith and Lourido conducted further experiments to understand SPARK’s role, and found evidence that the protein was involved in the release of calcium in the cell that is required for a parasite to enter or leave a host cell.
“The thing I found very interesting about SPARK is that it’s a kinase that’s very different from the analogous kinase in other model organisms, but is conserved throughout all of the apicomplexan phylum,” Smith said. “That’s the phylum that includes Toxoplasma and a bunch of other single-celled parasites like Plasmodium, which is the malaria parasite.”
Because SPARK is far different from its human analog and essential to the parasite’s life cycle, a SPARK-specific kinase inhibitor could be used to treat toxoplasmosis by killing the parasite without affecting the patient. “The hope would be that you can target SPARK and inhibit it without hitting mammalian kinases,” Smith said. “It’s easy enough to design something that kills a cell, but the trick is only killing parasites and not your own cells.”
In the future, the researchers hope to turn their new screening method to other families of genes, such as transcription factors, to understand their function in the parasites. “Our results have been quite encouraging in that we think this method will be scalable, and we can target larger gene sets in the future,” Smith said. “I think the ultimate end goal would be to do the whole genome.”
“There’s this whole universe of parasite proteins that we know so little about, where this type of analysis will be incredibly insightful.” Lourido said. “We’re really very excited about scaling it up further.
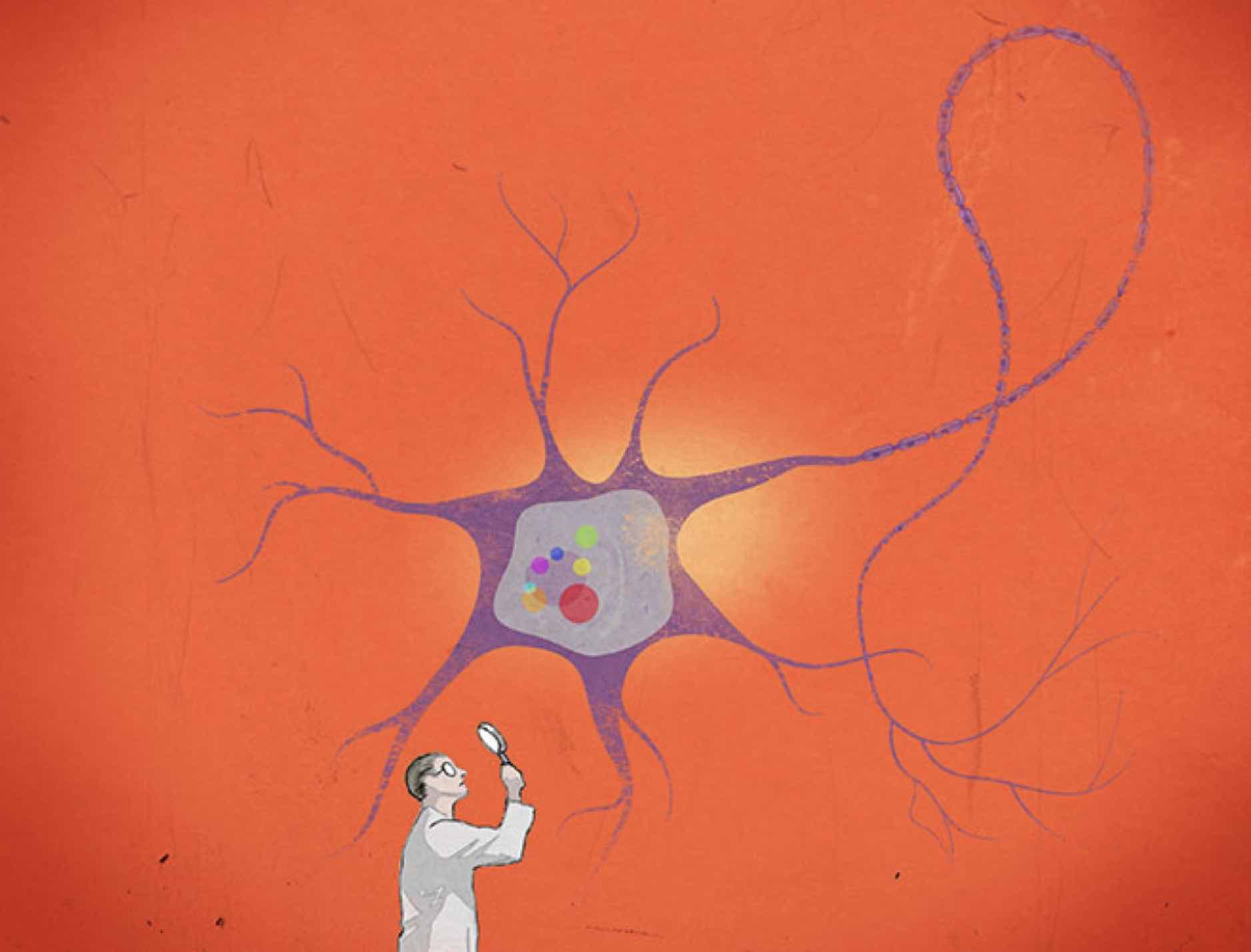
Greta Friar | Whitehead Institute
April 18, 2022
Drug overdose, mostly from opioid use, is the leading cause of accidental death in the United States. Prior studies of twins have revealed that genetics play a key role in opioid use disorder. Researchers know that a mixture of genetic and environmental risk factors contribute to heritability of the disorder, but identifying the specific risk factors is challenging. Opioid use disorder is complex, so instead of one or a few genes causing the disorder, there may be many contributing factors that can combine in different ways. Researchers want to understand which genes contribute to opioid use disorder because this will lead to a better understanding of its underlying biology and could help identify people who will be most at risk if exposed to opioids, enabling researchers, health care providers, and social services to develop strategies for prevention, treatment, and support.
The usual approach for finding genes associated with disease risk is to do a genome wide association study, which compares the genetics of many people to identify patterns in different gene versions occurring in association with a disease. This approach is being used to look at opioid use disorder, but requires many more patient samples than are currently available to reach clear conclusions. Researchers from multiple research universities and institutes, including Whitehead Institute Member Olivia Corradin and her former PhD advisor, Case Western Reserve University Professor Peter Scacheri; as well as Icahn School of Medicine Professor Schahram Akbarian; Eric O. Johnson, a distinguished fellow at RTI International; Dr. Kiran C. Patel College of Allopathic Medicine at Nova South Eastern University Professor Deborah C. Mash; and Richard Sallari of Axiotl, Inc., developed a shortcut for identifying genes that are associated with opioid use disorder and may contribute to it using only a small number of patient samples. Genome wide studies may require hundreds of thousands of samples, but this new method, described in their research published in the journal Molecular Psychiatry on March 17, uses only around 100 samples—51 cases and 51 controls—to narrow in on five candidate genes.
“With this work, we think we’re only seeing the tip of the iceberg of the complex, diverse factors contributing to opioid overdose,” says Corradin, who is also an assistant professor of biology at the Massachusetts Institute of Technology. “However, we hope our findings can help prioritize genes for further study, to speed up the identification of risk markers and possible therapeutic targets.”
In order to learn more about the underlying biology of opioid use disorder, the researchers analyzed brain tissue samples from people who had died of opioid overdoses and compared them with samples from people with no known opioid use history who died of other accidental causes. They specifically looked at neurons from the dorsolateral prefrontal cortex, an area of the brain known to play important roles in addiction. Instead of analyzing the genes in these cells directly, the researchers instead looked at the regulators of the genes’ activity, and searched for changes in these regulators that could point them to genes of interest.
To identify a gene, first map its community
Genes have DNA regions, often close to the gene, that can ratchet up and down the gene’s expression, or the strength of its activity in certain cells. Researchers have only recently been able to map the three-dimensional organization of DNA in a cell well enough to identify all of the regulators that are close to and acting upon target genes. Corradin and her collaborators call a gene’s collection of close regulatory elements its “plexus.” Their approach finds genes of interest by searching for patterns of variation across each gene’s entire plexus, which can be easier to spot with a small sample size.
The patterns that the researchers look for in a plexus are epigenetic changes: differences in the chemical tags that affect regulatory DNA and in turn, modify the expression of the regulators’ target gene. In this case, the researchers looked at a type of epigenetic tag called H3K27 acetylation, which is linked to increases in the activity of regulatory regions. They found nearly 400 locations in the DNA that consistently had less H3K27 acetylation in the brains of people who died of opioid overdose, which would lower activity of target genes. They also identified under-acetylated DNA locations that were often specific to individuals rather than uniform across all opioid overdose cases. The researchers then looked at how many of those locations belonged to regulatory elements in the same plexus. Surprisingly, these individual-specific changes often occurred within the same gene’s plexus. A gene whose plexus had been heavily affected as a collective was flagged as a possible contributor to opioid use disorder.
“We know that the factors that contribute to opioid use disorder are numerous, and that it’s an extremely complex disease that by definition is going to be extremely heterogeneous,” Scacheri says. “The idea was to figure out an approach that embraces that heterogeneity, and then try to spot the themes within it.”
Using this approach, the researchers identified five candidate genes, ASTN2, KCNMA1, DUSP4, GABBR2, and ENOX1. One of the genes, ASTN2, is related to pain tolerance, while KCNMA1, DUSP4, and GABBR2 are active in signaling pathways that have been linked more broadly to addiction. Follow up experiments can confirm whether these genes contribute to opioid use disorder.
The five genes and their plexi are also involved in the heritability of generalized anxiety disorder, metrics of tolerance for risk-taking, and educational attainment. Heritability of these traits and opioid use disorder have previously been found to coincide, and people with opioid use disorder often also have generalized anxiety. Furthermore, heritability of these traits and opioid use disorder all have been associated with early childhood adversity. These connections suggest the possibility that early childhood adversity could be contributing to the epigenetic changes observed by the researchers in the brains of people who died of opioid overdose—a useful hypothesis for further research.
The researchers hope that these results will provide some insights into the genetics and neurobiology of opioid use disorder. They are interested in moving their research forward in several ways: they would like to see if they can identify more candidate genes by increasing their sample number, examine different parts of the brain and different cell types, and further analyze the genes already identified. They also hope that their results demonstrate the potency of their approach, which was able to discern useful patterns and identify candidate genes from the neurons of only 51 cases.
“We’re trying a different approach here that relies on this idea of convergence and leverages our understanding of the three-dimensional architecture of DNA, and I hope this approach will be applied to further our understanding of all sorts of complex diseases,” Scacheri says.
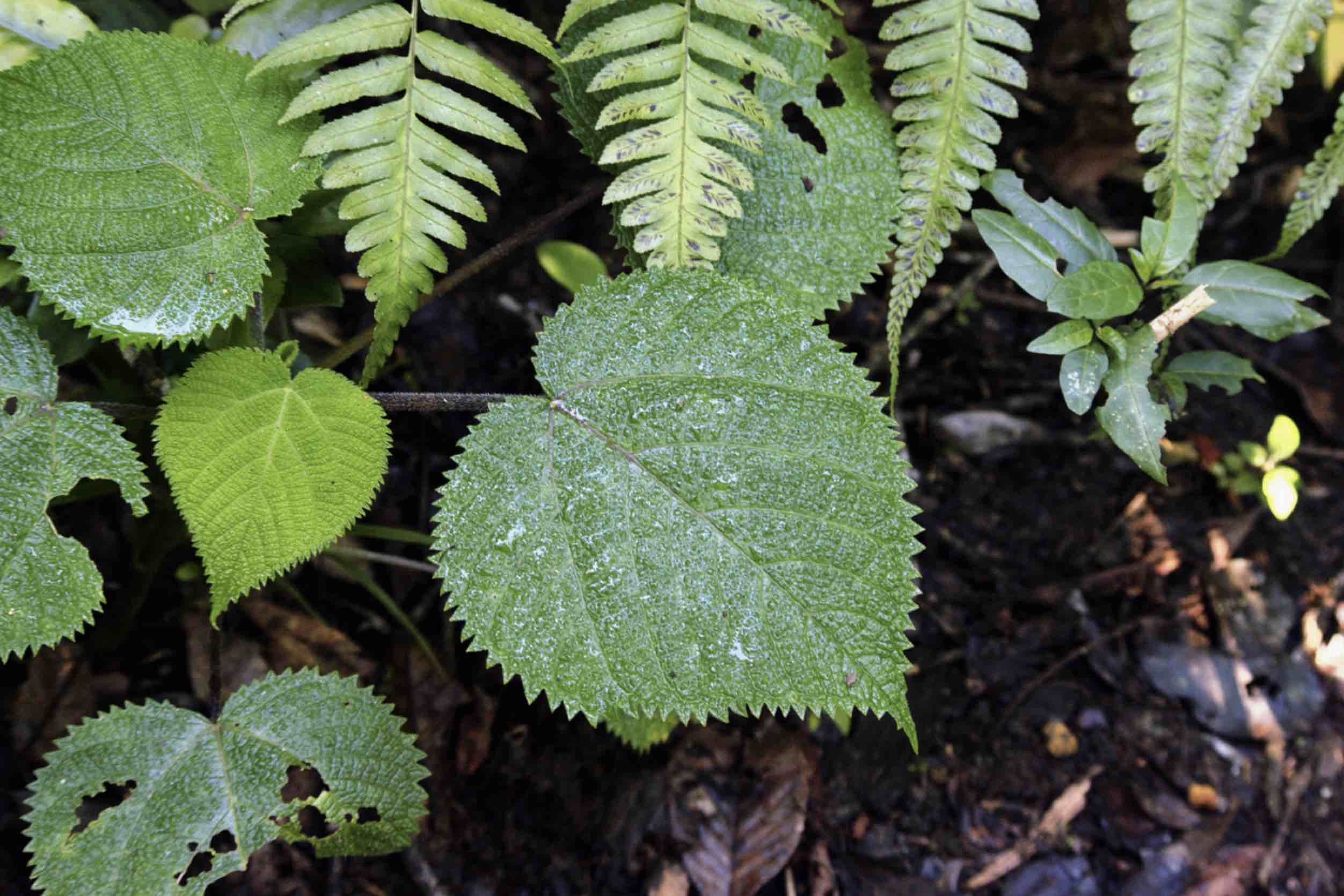
Eva Frederick | Whitehead Institute
April 20, 2022
The Australian stinging tree (Dendrocnide moroides) is a plant that many people avoid at all costs. The tree, which is a member of the nettle family, is covered in thin silicon needles laced with one of nature’s most excruciating toxins, a compound called moroidin. “It’s notorious for causing extreme pain, which lingers for a very long time,” said Whitehead Institute Member Jing-Ke Weng.
There’s another side to moroidin, though; in addition to causing pain, the compound binds to cells’ cytoskeletons, preventing them from dividing, which makes moroidin a promising candidate for chemotherapy drugs.
Harvesting enough of the chemical to study has proven difficult, for obvious reasons. Now, in a paper published April 19 in the Journal of the American Chemical Society, Weng, who is also an associate professor of biology at the Massachusetts Institute of Technology (MIT) and former postdoc Roland Kersten, now an assistant professor at the University of Michigan College of Pharmacy, present the first published method to biosynthesize moroidin within the tissues of harmless plants such as tobacco, facilitating research on the compound’s utility for cancer treatments.
Taking a leaf out of plants’ book to create peptides
Moroidin is a bicyclic peptide — a type of molecule made up of building blocks called amino acids and circularized to contain two connected rings. For synthetic chemists, moroidin has proved nearly impossible to synthesize due to its complex chemical structure. Weng and Kersten wanted to dig deeper into what methods the plants were using to create this molecule.
In plant cells, cyclic peptides are made from specific precursor proteins synthesized by the ribosome, the macromolecular machine that produces proteins by translating messenger RNAs. After leaving the ribosome, these precursor proteins are further processed by other enzymes in the cell to give rise to the final cyclic peptides. In 2018, Weng and Kersten had elucidated the biosynthetic mechanism of another type of plant peptides called lyciumins, first found in the goji berry plant, which gave them some insight into how post-translational modifications might play a role in creating different types of plant peptide chemistry. “We learned a lot about the principal elements of this system by studying lyciumins,” said Weng.
When they began to look into how moroidin was synthesized, the researchers found a few other plants, such as Kerria japonica and Celosia argentea, also produce peptides with similar chemistry to moroidin. “That really gave us the very critical insight that this is a new class of peptides,” Weng said.
Weng and Kersten previously learned that the BURP domain, which is part of the precursor proteins for lyciumins and several other plant cyclic peptides, catalyzes key reactions involved in the peptide ring formation. They found that the BURP domain was present in the precursor proteins for moroidins in Kerria japonica, and seemed to be essential for creating the two-ring structure of the molecules. The BURP domain creates ring chemistry when in the presence of copper, and when the researchers incubated the moroidin precursor protein with copper chloride in the lab together with other downstream proteolytic enzymes, they were able to create moroidin-like peptides.
With this information, they were able to produce a variety of moroidin analogs in tobacco plants by transgenically expressing the moroidin precursor gene of Kerria japonica and varying the core motif sequence corresponding to moroidin peptides. “We show that you can produce the same moroidin chemistry in a different host plant,” Weng said. “Tobacco itself is easier to be farmed on a large scale, and we also think in the future we can derive a plant cell line from the existing tobacco cell lines that we put in the moroidin precursor peptide, then we can use the cell line to produce the molecule, which really enables us to scale up for medicine production.”
Future use of moroidin
Moroidin’s anti-cancer property is due, at least in part, to the compound’s unique structure that allows it to bind to a protein called tubulin. Tubulin forms a skeletal system for living cells, and provides the means by which cells separate their chromosomes as they prepare to divide. Currently, two existing anti-cancer drugs, vincristine and paclitaxel, work by binding tubulin. These two compounds are derived from plants as well (the Madagascar periwinkle and Pacific yew tree, respectively).
In their new work, Weng and Kersten synthesized a moroidin analog called celogentin C. They tested its anti-cancer activity against a human lung cancer cell line, and found that the compound was toxic to the cancer cells. Their new study also suggests potentially new anti-cancer mechanisms specific to this lung cancer cell line in addition to tubulin inhibition.
In the past, researchers have run into issues when trying to create effective drugs from peptides. “There are two major challenges for peptides as medicine,” Weng said. “For one thing they are not very stable in vivo, and for another they are not very bioavailable and don’t readily pass the membrane of a cell.”
But cyclic peptides like moroidin and its analogs are a bit different. “These peptides essentially evolve to be drug-like,” Weng said. “In the case of the Australian stinging tree, the peptides are present because the plants want to deter any animals that want to eat the leaves. So over millions of years of evolution these plants eventually figured out a way to construct these specific cyclic peptides that are stable, bioavailable and can get to the animal that is trying to eat the plants.”
It’s likely that the painful reaction that occurs when moroidin enters the body through a sting from the tree would not be an issue in traditional methods of administering chemotherapy. “The pain is really caused if you get injections of the compound into the skin,” Weng said. “If you take it orally or intravenously, your body will most likely not sense the pain.”
Somewhat counterintuitively, the compound could also be used as a pain reliever. “If something causes pain, you can sometimes use that as an anti-pain medicine,” Weng said. “You could essentially exhaust the pain receptors, or if you alter the structure a little bit, you could turn an agonist into an antagonist and potentially block the pain.”
On a more fundamental level, moroidin could help researchers study pain receptors. “We don’t know exactly why being stung by the stinging tree produces that enormous amount of pain, and there may be additional pain receptors people haven’t identified,” Weng said. “Being able to synthesize moroidin provides a chemical probe that allows us to study this unknown pain perception in humans.”
In the future, the researchers hope to create analogs of moroidin to study, and hopefully create an optimal version for use in cancer therapy. “We want to generate a library of moroidin-like peptides,” Weng said. “We’ve done this for lyciumins, and since the initial moroidins are anti-tubulin molecules, we can use this system to find an improved version that binds to tubulin even tighter and contains other pharmacological properties making it suitable to be used as a therapeutic.