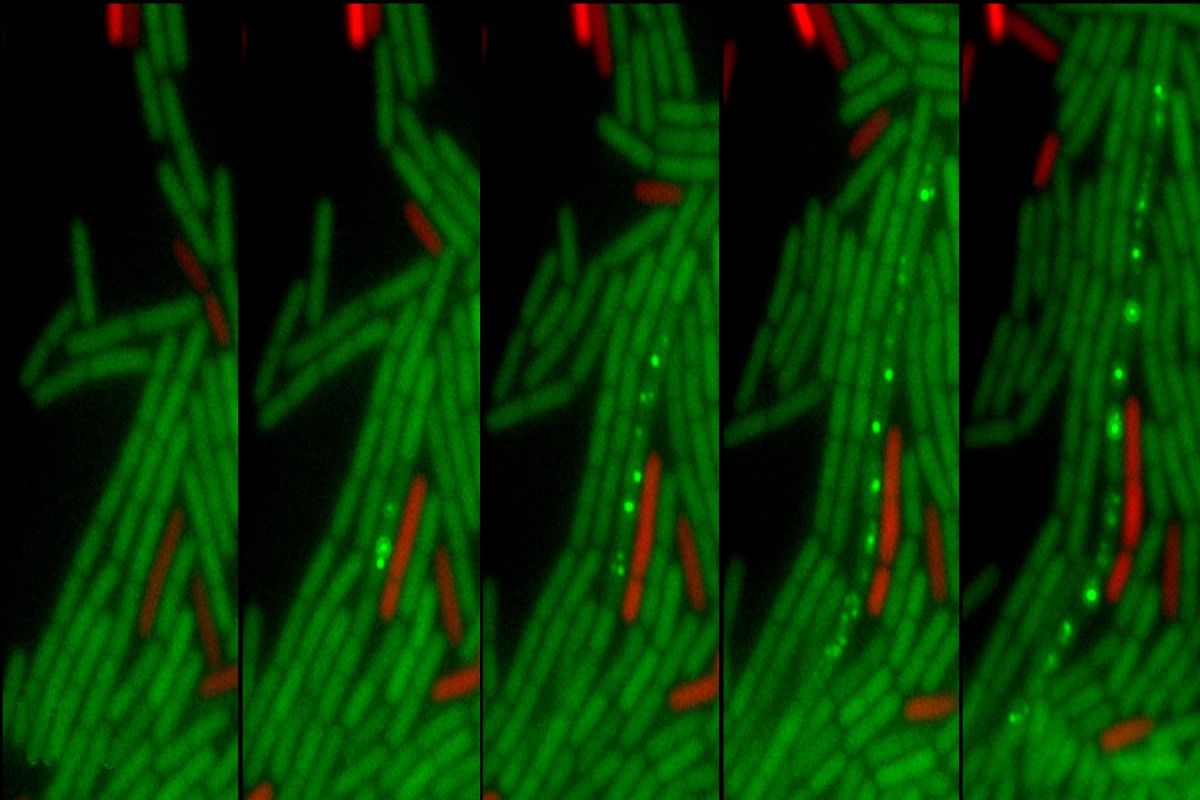
Researchers identify a strategy to prevent mobile genetic elements from breaching the bacterial cell wall.
Raleigh McElvery | Department of Biology
August 6, 2019
We receive half of our genes from each biological parent, so there’s no avoiding inheriting a blend of characteristics from both. Yet, for single-celled organisms like bacteria that reproduce by splitting into two identical cells, injecting variety into the gene pool isn’t so easy. Random mutations add some diversity, but there’s a much faster way for bacteria to reshuffle their genes and confer evolutionary advantages like antibiotic resistance or pathogenicity.
Known as horizontal gene transfer, this process permits bacteria to pass pieces of DNA to their peers, in some cases allowing those genes to be integrated into the recipient’s genome and passed down to the next generation.
The Grossman lab in the MIT Department of Biology studies one class of mobile DNA, known as integrative and conjugative elements (ICEs). While ICEs contain genes that can be beneficial to the recipient bacterium, there’s also a catch — receiving a duplicate copy of an ICE is wasteful, and possibly lethal. The biologists recently uncovered a new system by which one particular ICE, ICEBs1, blocks a donor bacterium from delivering a second, potentially deadly copy.
“Understanding how these elements function and how they’re regulated will allow us to determine what drives microbial evolution,” says Alan Grossman, department head and senior author on the study. “These findings not only provide insight into how bacteria block unwanted genetic transfer, but also how we might eventually engineer this system to our own advantage.”
Former graduate student Monika Avello PhD ’18 and current graduate student Kathleen Davis are co-first authors on the study, which appeared online in Molecular Microbiology on July 30.
Checks and balances
Although plasmids are perhaps the best-known mediators of horizontal transfer, ICEs not only outnumber plasmids in most bacterial species, they also come with their own tools to exit the donor, enter the recipient, and integrate themselves into the recipient’s chromosome. Once the donor bacterium makes contact with the recipient, the machinery encoded by the ICE can pump the ICE DNA from one cell to the other through a tiny channel.
For horizontal transfer to proceed, there are physical barriers to overcome, especially in so-called Gram-positive bacteria, which boast thicker cell walls than their Gram-negative counterparts, despite being less widely studied. According to Davis, the transfer machinery essentially has to “punch a hole” through the recipient cell. “It’s a rough ride and a waste of energy for the recipient if that cell already contains an ICE with a specific set of genes,” she says.
Sure, ICEs are “selfish bits of DNA” that persist by spreading themselves as widely as possible, but in order to do so they must not interfere with their host cell’s ability to survive. As Avello explains, ICEs can’t just disseminate their DNA “without certain checks and balances.”
“There comes a point where this transfer comes at a cost to the bacteria or doesn’t make sense for the element,” she says. “This study is beginning to get at the question of when, why, and how ICEs might want to block transfer.”
The Grossman lab works in the Gram-positive Bacillus subtilis, and had previously discovered two mechanisms by which ICEBs1 could prevent redundant transfer before it becomes lethal. The first, cell-cell signaling, involves the ICE in the recipient cell releasing a chemical cue that prohibits the donor’s transfer machinery from being assembled. The second, immunity, initiates if the duplicate copy is already inside the cell, and prevents the replicate from being integrated into the chromosome.
However, when the researchers tried eliminating both fail-safes simultaneously, rather than re-instating ICE transfer as they expected, the bacteria still managed to obstruct the duplicate copy. ICEBs1 seemed to have a third blocking strategy, but what might it be?
The third tactic
In this most recent study, they’ve identified the mysterious blocking mechanism as a type of “entry exclusion,” whereby the ICE in the recipient cell encodes molecular machinery that physically prevents the second copy from breaching the cell wall. Scientists had observed other mobile genetic elements capable of exclusion, but this was the first time anyone had witnessed this phenomenon for an ICE from Gram-positive bacteria, according to Avello.
The Grossman lab determined that this exclusion mechanism comes down to two key proteins. Avello identified the first protein, YddJ, expressed by the ICEBs1 in the recipient bacterium, forming a “protective coating” on the outside of the cell and blocking a second ICE from entering.
But the biologists still didn’t know which piece of transfer machinery YddJ was blocking, so Davis performed a screen and various genetic manipulations to pinpoint YddJ’s target. YddJ, it turned out, was obstructing another protein called ConG, which likely forms part of the transfer channel between the donor and recipient bacteria. Davis was surprised to find that, while Gram-negative ICEs encode a protein that’s quite similar to ConG, the Gram-negative YddJ equivalent is actually much different.
“This just goes to show that you can’t assume the transfer machinery in Gram-positive ICEs like ICEBs1 are the same as the well-studied Gram-negative ICEs,” she says.
The team concluded that ICEBs1 must have three different mechanisms to prevent duplicate transfer: the two they’d previously uncovered plus this new one, exclusion.
Cell-cell signaling allows a cell to spread the word to its neighbors that it already has a copy of ICEBs1, so there’s no need to bother assembling the transfer machinery. If this fails, exclusion kicks in to physically block the transfer machinery from penetrating the recipient cell. If that proves unsuccessful and the second copy enters the recipient, immunity will initiate and prevent the second copy from being integrated into the recipient’s chromosome.
“Each mechanism acts at a different step, because none of them alone are 100 percent effective,” Grossman says. “That’s why it’s helpful to have multiple mechanisms.”
They don’t know all the details of this transfer machinery just yet, he adds, but they do know that YddJ and ConG are key players.
“This initial description of the ICEBs1 exclusion system represents the first report that provides mechanistic insights into exclusion in Gram-positive bacteria, and one of only a few mechanistic studies of exclusion in any conjugation system,” says Gary Dunny, a professor of microbiology and immunology at the University of Minnesota who was not involved in the study. “This work is significant medically because ICEs can carry “cargo” genes such as those conferring antibiotic resistance, and also of importance to our basic understanding of horizontal gene transfer systems and how they evolve.”
As researchers continue to probe this blocking mechanism, it might be possible to leverage ICE exclusion to design bacteria with specific functions. For instance, they could engineer the gut microbiome and introduce beneficial genes to help with digestion. Or, one day, they could perhaps block horizontal gene transfer to combat antibiotic resistance.
“We had suspected that Gram-positive ICEs might be capable of exclusion, but we didn’t have proof before this,” Avello says. Now, researchers can start to speculate about how pathogenic Gram-positive species might control the movement of ICEs throughout a bacterial population, with possible ramifications for disease research.
This work was funded by research and predoctoral training grants from the National Institute of General Medical Sciences of the National Institutes of Health.